Power-to-X
Power-to-X (also P2X and P2Y) is a number of electricity conversion, energy storage, and reconversion pathways that use surplus electric power, typically during periods where fluctuating renewable energy generation exceeds load.[1][2] Power-to-X conversion technologies allow for the decoupling of power from the electricity sector for use in other sectors (such as transport or chemicals), possibly using power that has been provided by additional investments in generation.[1] The term power-to-x is widely used in Germany and may have originated there.
The X in the terminology can refer to one of the following: power-to-ammonia, power-to-chemicals, power-to-fuel, power-to-gas, power-to-heat, power-to-hydrogen, power-to-liquid, power-to-methane, power-to-mobility, power to food, power-to-power, and power-to-syngas.
Collectively power-to-X schemes which use surplus power fall under the heading of flexibility measures and are particularly useful in energy systems with high shares of renewable generation and/or with strong decarbonization targets.[1][2] A large number of pathways and technologies are encompassed by the term. In 2016 the German government funded a €30 million first-phase research project into power-to-X options.[3]
Electricity storage concepts
Surplus electric power can be converted to other forms of energy for storage and reconversion.[4][5][6][7] Direct current electrolysis (efficiency 80–85% at best) can be used to produce hydrogen which can, in turn, be converted to methane (CH4) via methanation.[4][8] Another possibility is converting the hydrogen, along with CO2 to methanol.[9] Both these fuels can be stored and used to produce electricity again, hours to months later. Reconversion technologies include gas turbines, CCGT plant, reciprocating engines and fuel cells. Power-to-power refers to the round-trip reconversion efficiency.[4] For hydrogen storage, the round-trip efficiency remains limited at 35–50%.[2] Electrolysis is expensive and power-to-gas processes need substantial full-load hours (say 30%) to be economic.[1] However, while round-trip conversion efficiency of power-to-power is lower than with batteries and electrolysis can be expensive, storage of the fuels themselves is quite inexpensive. This means that large amounts of energy can be stored for long periods of time with power-to-power, which is ideal for seasonal storage. This could be particularly useful for systems with high renewable penetration, since many areas have significant seasonal variability of solar, wind, and hydroelectric generation. Grid-dedicated battery storage is not normally considered a power-to-X concept.
Sector coupling concepts
Hydrogen and methane can also be used as downstream fuels, feed into the natural gas grid, or used to make or synthetic fuel.[10][11] Alternatively they can be used as a chemical feedstock, as can ammonia (NH
3).
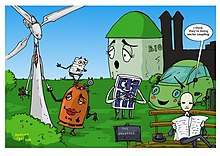
Power-to-heat involves contributing to the heat sector, either by resistance heating or via a heat pump. Resistance heaters have unity efficiency, and the corresponding coefficient of performance (COP) of heat pumps is 2–5.[4] Back-up immersion heating of both domestic hot water and district heating offers a cheap way of using surplus renewable energy and will often displace carbon-intensive fossil fuels for the task.[1] Large-scale heat pumps in district heating systems with thermal energy storage are an especially attractive option for power-to-heat: they offer exceptionally high efficiency for balancing excess wind and solar power, and they can be profitable investments.[12][13]
Power-to-mobility refers to the charging of battery electric vehicles (EV). Given the expected uptake of EVs, dedicated dispatch will be required. As vehicles are idle for most of the time, shifting the charging time can offer considerable flexibility: the charging window is a relatively long 8–12 hours, whereas the charging duration is around 90 minutes.[2] The EV batteries can also be discharged to the grid to make them work as electricity storage devices, but this causes additional wear to the battery.[2]
Heat pumps with hot water storage and electric vehicles have been found to have higher potential on reduction of CO
2 emissions and fossil fuel use than several other power-to-X or electricity storage schemes for using surplus wind and solar power.[4] However, while power-to-heat and power-to-mobility through electrification (heat pumps and electric vehicles) have a high emissions reduction potential, if the goal is a 100% clean system, there are some end uses which cannot be economically electrified. These end uses include long distance shipping (trucking, airplanes, barges) and high heat industrial processes. In these cases, using fuels synthesized from clean electricity might be the best option. Biofuels are another option, but they compete with agriculture for water and space.
According to the German concept of sector coupling interconnecting all the energy-using sectors will require the digitalisation and automation of numerous processes to synchronise supply and demand.[14]
See also
- Energy storage
- Grid energy storage
- Power-to-gas
- Power-to-heat
References
- acatech; Lepoldina; Akademienunion, eds. (2016). Flexibility concepts for the German power supply in 2050 : ensuring stability in the age of renewable energies (PDF). Berlin, Germany: acatech — National Academy of Science and Engineering. ISBN 978-3-8047-3549-1. Archived from the original (PDF) on 6 October 2016. Retrieved 10 June 2016.
- Lund, Peter D; Lindgren, Juuso; Mikkola, Jani; Salpakari, Jyri (2015). "Review of energy system flexibility measures to enable high levels of variable renewable electricity" (PDF). Renewable and Sustainable Energy Reviews. 45: 785–807. doi:10.1016/j.rser.2015.01.057.
- "Power-to-X: entering the energy transition with Kopernikus" (Press release). Aachen, Germany: RWTH Aachen. 5 April 2016. Retrieved 9 June 2016.
- Sternberg, André; Bardow, André (2015). "Power-to-What? — Environmental assessment of energy storage systems". Energy and Environmental Science. 8 (2): 389–400. doi:10.1039/c4ee03051f.
- Agora Energiewende (2014). Electricity storage in the German energy transition : analysis of the storage required in the power market, ancillary services market and the distribution grid (PDF). Berlin, Germany: Agora Energiewende. Retrieved 30 December 2018.
- Sterner, Michael; Eckert, Fabian; Thema, Martin; et al. (2014). Langzeitspeicher in der Energiewende — Präsentation [Long-term storage in the Energiewende — Presentation]. Regensburg, Germany: Forschungsstelle für Energienetze und Energiespeicher (FENES), OTH Regensburg. Retrieved 9 May 2016.
- Ausfelder, Florian; Beilmann, Christian; Bräuninger, Sigmar; Elsen, Reinhold; Hauptmeier, Erik; Heinzel, Angelika; Hoer, Renate; Koch, Wolfram; Mahlendorf, Falko; Metzelthin, Anja; Reuter, Martin; Schiebahn, Sebastian; Schwab, Ekkehard; Schüth, Ferdi; Stolten, Detlef; Teßmer, Gisa; Wagemann, Kurt; Ziegahn, Karl-Friedrich (May 2016). Energy storage systems: the contribution of chemistry — Position paper (PDF). Germany: Koordinierungskreis Chemische Energieforschung (Joint Working Group on Chemical Energy Research). ISBN 978-3-89746-183-3. Retrieved 9 June 2016.
- Pagliaro, Mario; Konstandopoulos, Athanasios G (15 June 2012). Solar Hydrogen: Fuel of the Future. Cambridge, United Kingdom: RSC Publishing. doi:10.1039/9781849733175. ISBN 978-1-84973-195-9.
- George Olah's renewable methanol plant
- König, Daniel Helmut; Baucks, Nadine; Kraaij, Gerard; Wörner, Antje (18–19 February 2014). "Entwicklung und Bewertung von Verfahrenskonzepten zur Speicherung von fluktuierenden erneuerbaren Energien in flüssigen Kohlenwasserstoffen" [Development and evaluation of process concepts for storing fluctuating renewable energy in liquid hydrocarbons]. Jahrestreffen der ProcessNet-Fachgruppe Energieverfahrenstechnik. Karlsruhe, Germany. Retrieved 9 May 2016.
- Foit, Severin; Eichel, Rüdiger-A; Vinke, Izaak C; de Haart, Lambertus GJ (1 October 2016). "Power-to-Syngas – an enabling technology for the transition of the energy system? Production of tailored synfuels and chemicals using renewably generated electricity". Angewandte Chemie International Edition. 56 (20): 5402–5411. doi:10.1002/anie.201607552. ISSN 1521-3773. PMID 27714905.
- Zakeri, Behnam; Rinne, Samuli; Syri, Sanna (31 March 2015). "Wind integration into energy systems with a high share of nuclear power – what are the compromises?". Energies. 8 (4): 2493–2527. doi:10.3390/en8042493. ISSN 1996-1073.
- Salpakari, Jyri; Mikkola, Jani; Lund, Peter D (2016). "Improved flexibility with large-scale variable renewable power in cities through optimal demand side management and power-to-heat conversion". Energy Conversion and Management. 126: 649–661. doi:10.1016/j.enconman.2016.08.041. ISSN 0196-8904.
- "Sector coupling - Shaping an integrated renewable energy system". Clean Energy Wire. 18 April 2018. Retrieved 6 March 2019.