Single-cell analysis
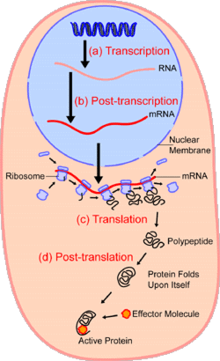
In the field of cellular biology, single-cell analysis is the study of genomics, transcriptomics, proteomics and metabolomics at the single cell level.[1][2] Due to the heterogeneity seen in both eukaryotic and prokaryotic cell populations, analyzing a single cell makes it possible to discover mechanisms not seen when studying a bulk population of cells.[3] Technologies such as fluorescence-activated cell sorting (FACS) have increased the throughput of single cell sorting, and increased the development of single cell analysis techniques. The development of new technologies are increasing our ability to sequence the genome, and transcriptome, of single cells, as well as to quantify their proteome and metabolome.[4][5][6] New developments in mass spectrometry techniques have become important analytical tools for proteomic and metabolomic analysis of single cells.[7][8] In situ sequencing and fluorescence in situ hybridization (FISH) do not require that cells be isolated and are increasingly being used for analysis of tissues.[9]
Single-cell isolation
Many single-cell analysis techniques require the isolation of individual cells. Methods currently used for single cell isolation include: serial dilution, micromanipulation, laser capture microdissection, FACS, microfluidics, manual picking, enzymatic digestion, and Raman tweezers.[10]
Manual single cell picking is a method is where cells in a suspension are viewed under a microscope, and individually picked using a micropipette.[11][12] Raman tweezers is a technique where Raman spectroscopy is combined with optical tweezers, which uses a laser beam to trap, and manipulate cells.[13]
The development of hydrodynamic-based microfluidic biochips has been increasing over the years. In this technique, the cells or particles are trapped in a particular region for single cell analysis (SCA) usually without any application of external force fields such as optical, electrical, magnetic or acoustic. There is a need to explore the insights of SCA in the cell's natural state and development of these techniques is highly essential for that study. Researchers have highlighted the vast potential field that needs to be explored to develop biochip devices to suit market/researcher demands. Hydrodynamic microfluidics facilitates the development of passive lab-on-chip applications. A latest review gives an account of the recent advances in this field, along with their mechanisms, methods and applications.[14]
Genomics
Techniques
Single-cell genomics is heavily dependent on increasing the copies of DNA found in the cell so there is enough to be sequenced. This has led to the development of strategies for whole genome amplification (WGA). One widely adopted WGA techniques is called degenerate oligonucleotide–primed polymerase chain reaction (DOP-PCR). This method uses the well established DNA amplification method PCR to try and amplify the entire genome using a large set of primers. Although simple, this method has been shown to have very low genome coverage. An improvement on DOP-PCR is Multiple displacement amplification (MDA), which uses random primers and a high fidelity enzyme, usually Φ29 DNA polymerase, to accomplish the amplification of larger fragments and greater genome coverage than DOP-PCR. Despite these improvement MDA still has a sequence dependent bias (certain parts of the genome are amplified more than others because of their sequence). The method shown to largely avoid the bias seen in DOP-PCR and MDA is Multiple Annealing and Looping–Based Amplification Cycles (MALBAC). Bias in this system is reduced by only copying off the original DNA strand instead of making copies of copies. The main draw backs to using MALBA, is it has reduced accuracy compared to DOP-PCR and MDA due to the enzyme used to copy the DNA.[4] Once amplified using any of the above techniques, the DNA is sequenced using next-generation sequencing (NGS).
Purpose
There are two major applications to studying the genome at the single cell level. One application is to track the changes that occur in bacterial populations, where phenotypic differences are often seen. These differences are missed by bulk sequencing of a population, but can be observed in single cell sequencing.[15] The second major application is to study the genetic evolution of cancer. Since cancer cells are constantly mutating it is of great interest to see how cancers evolve at the genetic level. These patterns of mutation can be observed using single cell sequencing.[16]
Transcriptomics
Techniques
Single-cell transcriptomics uses sequencing techniques similar to single cell genomics or direct detection using fluorescence in situ hybridization. The first step in quantifying the transcriptome is to convert RNA to cDNA using reverse transcriptase so that the contents of the cell can be sequenced using NGS methods as was done in genomics. Once converted, there is not enough cDNA to be sequenced so the same DNA amplification techniques discussed in single cell genomics are applied to the cDNA to make sequencing possible.[16] Alternately, fluorescent compounds attached to RNA hybridization probes are used to identify specific sequences and sequential application of different RNA probes will build up a comprehensive transcriptome.[17][18]
Purpose
The purpose of single cell transcriptomics is to determine what genes are being expressed in each cell. The transcriptome is often used to quantify the gene expression instead of the proteome because of the difficulty currently associated with amplifying protein levels.[16]
There are three major reasons gene expression has been studied using this technique: to study gene dynamics, RNA splicing, and cell typing. Gene dynamics are usually studied to determine what changes in gene expression effect different cell characteristics. For example, this type of transcriptomic analysis has often been used to study embryonic development. RNA splicing studies are focused on understanding the regulation of different transcript isoforms. Single cell transcriptomics has also been used for cell typing, where the genes expressed in a cell are used to identify types of cells. The main goal in cell typing is to find a way to determine the identity of cells that don't have known genetic markers.[16]
Proteomics
Techniques
There are two major approaches to single-cell proteomics: antibody based methods, and mass spectroscopy based methods.
Antibody–based methods
The antibody based methods use designed antibodies to bind to proteins of interest. These antibodies can be bound to fluorescent molecules such as quantum dots or isotopes that can be resolved by mass spectrometry. Since different colored quantum dots or different isotopes are attached to different antibodies it is possible to identify multiple different proteins in a single cell. Quantum dots to be washed off of the antibodies without damaging the sample, making it possible to do multiple rounds of protein quantification using this method on the same sample.[19] Rare metal isotopes attached to antibodies, not normally found in cells or tissues can be detected by mass spectrometry for simultaneous and sensitive identification of proteins.[20]
Another antibody based method converts protein levels to DNA levels. The conversion to DNA makes it possible to amplify protein levels and use NGS to quantify proteins. To do this, two antibodies are designed for each protein needed to be quantified. The two antibodies are then modified to have single stranded DNA connected to them that are complimentary. When the two antibodies bind to a protein the complimentary strands will anneal and produce a double stranded piece of DNA that can then be amplified using PCR. Each pair of antibodies designed for one protein is tagged with a different DNA sequence. The DNA amplified from PCR can then be sequenced, and the protein levels quantified.[21]
Mass spectroscopy–based methods
In mass spectroscopy based proteomics there are three major steps needed for peptide identification: sample preparation, separation of peptides, and identification of peptides. Several groups have focused on oocytes or very early cleavage-stage cells since these cells are unusually large and provide enough material for analysis.[22][23][24] Another approach, single cell proteomics by mass spectrometry (SCoPE-MS) has quantified thousands of proteins in mammalian cells with typical cell sizes (diameter of 10-15 μm) by combining carrier-cells and labeling.[25][26][27] Multiple methods exist to isolate the peptides for analysis. These include using filter aided sample preparation, the use of magnetic beads, or using a series of reagents and centrifuging steps.[28][22][24] The separation of differently sized proteins can be accomplished by using capillary electrophoresis (CE) or liquid chromatograph (LC) (using liquid chromatography with mass spectroscopy is also known as LC-MS).[22][23][24][25] This step gives order to the peptides before quantification using tandem mass-spectroscopy (MS/MS). The major difference between quantification methods is some use labels on the peptides such as tandem mass tags (TMT) or dimethyl labels which are used to identify which cell a certain protein came from (proteins coming from each cell have a different label) while others use not labels (quantify cells individually). The mass spectroscopy data is then analyzed by running data through databases that convert the information about peptides identified to quantification of protein levels.[22][23][24][25][29] These methods are very similar to those used to quantify the proteome of bulk cells, with modifications to accommodate the very small sample volume.[26] Improvements in sample preparation, mass-spec methods and data analysis can increase the sensitivity and throughput by orders of magnitude.[30]
Purpose
The purpose of studying the proteome is to better understand the activity of cells at the single cells level. Since proteins are responsible for determining how the cell acts, understanding the proteome of single cell gives the best understanding of how a cell operates, and how gene expression changes in a cell due to different environmental stimuli. Although transcriptomics has the same purpose as proteomics it is not as accurate at determining gene expression in cells as it does not take into account post-transcriptional regulation.[5] Transcriptomics is still important as studying the difference between RNA levels and protein levels could give insight on which genes are post-transcriptionally regulated.
Metabolomics
Techniques
There are four major methods used to quantify the metabolome of single cells, they are: fluorescence–based detection, fluorescence biosensors, FRET biosensors, and mass spectroscopy. The first three methods listed use fluorescence microscopy to detect molecules in a cell. Usually these assays use small fluorescent tags attached to molecules of interest, however this has been shown be too invasive for single cell metabolomics, and alters the activity of the metabolites. The current solution to this problem is to use fluorescent proteins which will act as metabolite detectors, fluorescing when ever they bind to a metabolite of interest.[31]
Mass spectroscopy is becoming the most frequently used method for single cell metabolomics. Its advantages are that there is no need to develop fluorescent proteins for all molecules of interest, and is capable of detecting metabolites in the femtomole range.[8] Similar to the methods discussed in proteomics, there has also been success in combining mass spectroscopy with separation techniques such as capillary electrophoresis to quantify metabolites. This method is also capable of detecting metabolites present in femtomole concentrations.[31] Another method utilizing capillary microsampling combined with mass spectrometry with ion mobility separation has been demonstrated to enhance the molecular coverage and ion separation for single cell metabolomics.[12][32]
Purpose
The purpose of single cell metabolomics is to gain a better understanding at the molecular level of major biological topics such as: cancer, stem cells, aging, as well as the development of drug resistance. In general the focus of metabolomics is mostly on understanding how cells deal with environmental stresses at the molecular level, and to give a more dynamic understanding of cellular functions.[31]
See also
References
- ↑ Wang D, Bodovitz S (June 2010). "Single cell analysis: the new frontier in 'omics'". Trends in Biotechnology. 28 (6): 281–90. doi:10.1016/j.tibtech.2010.03.002. PMC 2876223. PMID 20434785.
- ↑ Habibi I, Cheong R, Lipniacki T, Levchenko A, Emamian ES, Abdi A (April 2017). "Computation and measurement of cell decision making errors using single cell data". PLoS Computational Biology. 13 (4): e1005436. doi:10.1371/journal.pcbi.1005436. PMC 5397092. PMID 28379950.
- ↑ Altschuler SJ, Wu LF (May 2010). "Cellular heterogeneity: do differences make a difference?". Cell. 141 (4): 559–63. doi:10.1016/j.cell.2010.04.033. PMC 2918286. PMID 20478246.
- 1 2 Huang L, Ma F, Chapman A, Lu S, Xie XS (2015). "Single-Cell Whole-Genome Amplification and Sequencing: Methodology and Applications". Annual Review of Genomics and Human Genetics. 16 (1): 79–102. doi:10.1146/annurev-genom-090413-025352. PMID 26077818.
- 1 2 Wu AR, Wang J, Streets AM, Huang Y (June 2017). "Single-Cell Transcriptional Analysis". Annual Review of Analytical Chemistry. 10 (1): 439–462. doi:10.1146/annurev-anchem-061516-045228. PMID 28301747.
- ↑ Tsioris K, Torres AJ, Douce TB, Love JC (2014). "A new toolbox for assessing single cells". Annual Review of Chemical and Biomolecular Engineering. 5: 455–77. doi:10.1146/annurev-chembioeng-060713-035958. PMC 4309009. PMID 24910919.
- ↑ Comi TJ, Do TD, Rubakhin SS, Sweedler JV (March 2017). "Categorizing Cells on the Basis of their Chemical Profiles: Progress in Single-Cell Mass Spectrometry". Journal of the American Chemical Society. 139 (11): 3920–3929. doi:10.1021/jacs.6b12822. PMID 28135079.
- 1 2 Zhang L, Vertes A (April 2018). "Single-Cell Mass Spectrometry Approaches to Explore Cellular Heterogeneity". Angewandte Chemie. 57 (17): 4466–4477. doi:10.1002/anie.201709719. PMID 29218763.
- ↑ Lee JH (July 2017). "De Novo Gene Expression Reconstruction in Space". Trends in Molecular Medicine. 23 (7): 583–593. doi:10.1016/j.molmed.2017.05.004. PMC 5514424. PMID 28571832.
- ↑ Yilmaz S, Singh AK (June 2012). "Single cell genome sequencing". Current Opinion in Biotechnology. Energy biotechnology • Environmental biotechnology. 23 (3): 437–43. doi:10.1016/j.copbio.2011.11.018. PMC 3318999. PMID 22154471.
- ↑ Gross A, Schoendube J, Zimmermann S, Steeb M, Zengerle R, Koltay P (July 2015). "Technologies for Single-Cell Isolation". International Journal of Molecular Sciences. 16 (8): 16897–919. doi:10.3390/ijms160816897. PMC 4581176. PMID 26213926.
- 1 2 Zhang L, Vertes A (October 2015). "Energy Charge, Redox State, and Metabolite Turnover in Single Human Hepatocytes Revealed by Capillary Microsampling Mass Spectrometry". Analytical Chemistry. 87 (20): 10397–405. doi:10.1021/acs.analchem.5b02502. PMID 26398405.
- ↑ Faria E, Gardner P (2012-01-01). Lindström S, Andersson-Svahn H, eds. Single-Cell Analysis. Methods in Molecular Biology. Humana Press. pp. 151–167. doi:10.1007/978-1-61779-567-1_12. ISBN 9781617795664.
- ↑ Narayanamurthy V, Nagarajan S, Khan AY, Samsuri F, Sridhar TM (2017-06-30). "Microfluidic hydrodynamic trapping for single cell analysis: mechanisms, methods and applications". Analytical Methods. 9 (25): 3751–3772. doi:10.1039/C7AY00656J. ISSN 1759-9679.
- ↑ Kalisky T, Quake SR (April 2011). "Single-cell genomics". Nature Methods. 8 (4): 311–4. doi:10.1038/nmeth0411-311. PMID 21451520.
- 1 2 3 4 Wang D, Bodovitz S (June 2010). "Single cell analysis: the new frontier in 'omics'". Trends in Biotechnology. 28 (6): 281–90. doi:10.1016/j.tibtech.2010.03.002. PMC 2876223. PMID 20434785.
- ↑ Lubeck E, Coskun AF, Zhiyentayev T, Ahmad M, Cai L (April 2014). "Single-cell in situ RNA profiling by sequential hybridization". Nature Methods. 11 (4): 360–1. doi:10.1038/nmeth.2892. PMC 4085791. PMID 24681720.
- ↑ Chen KH, Boettiger AN, Moffitt JR, Wang S, Zhuang X (April 2015). "RNA imaging. Spatially resolved, highly multiplexed RNA profiling in single cells". Science. 348 (6233): aaa6090. doi:10.1126/science.aaa6090. PMC 4662681. PMID 25858977.
- ↑ Zrazhevskiy P, True LD, Gao X (October 2013). "Multicolor multicycle molecular profiling with quantum dots for single-cell analysis". Nature Protocols. 8 (10): 1852–69. doi:10.1038/nprot.2013.112. PMC 4108347. PMID 24008381.
- ↑ Nair N, Mei HE, Chen SY, Hale M, Nolan GP, Maecker HT, Genovese M, Fathman CG, Whiting CC (May 2015). "Mass cytometry as a platform for the discovery of cellular biomarkers to guide effective rheumatic disease therapy". Arthritis Research & Therapy. 17: 127. doi:10.1186/s13075-015-0644-z. PMC 4436107. PMID 25981462.
- ↑ Gong H, Holcomb I, Ooi A, Wang X, Majonis D, Unger MA, Ramakrishnan R (January 2016). "Simple Method To Prepare Oligonucleotide-Conjugated Antibodies and Its Application in Multiplex Protein Detection in Single Cells". Bioconjugate Chemistry. 27 (1): 217–25. doi:10.1021/acs.bioconjchem.5b00613. PMID 26689321.
- 1 2 3 4 Lombard-Banek C, Reddy S, Moody SA, Nemes P (August 2016). "Label-free Quantification of Proteins in Single Embryonic Cells with Neural Fate in the Cleavage-Stage Frog (Xenopus laevis) Embryo using Capillary Electrophoresis Electrospray Ionization High-Resolution Mass Spectrometry (CE-ESI-HRMS)". Molecular & Cellular Proteomics. 15 (8): 2756–68. doi:10.1074/mcp.M115.057760. PMC 4974349. PMID 27317400.
- 1 2 3 Sun L, Dubiak KM, Peuchen EH, Zhang Z, Zhu G, Huber PW, Dovichi NJ (July 2016). "Single Cell Proteomics Using Frog (Xenopus laevis) Blastomeres Isolated from Early Stage Embryos, Which Form a Geometric Progression in Protein Content". Analytical Chemistry. 88 (13): 6653–7. doi:10.1021/acs.analchem.6b01921. PMC 4940028. PMID 27314579.
- 1 2 3 4 Virant-Klun I, Leicht S, Hughes C, Krijgsveld J (August 2016). "Identification of Maturation-Specific Proteins by Single-Cell Proteomics of Human Oocytes". Molecular & Cellular Proteomics. 15 (8): 2616–27. doi:10.1074/mcp.M115.056887. PMC 4974340. PMID 27215607.
- 1 2 3 Budnik, Bogdan; Levy, Ezra; Slavov, Nikolai (2017-03-15). "Mass-spectrometry of single mammalian cells quantifies proteome heterogeneity during cell differentiation". bioRxiv 102681.
- 1 2 "SCoPE-MS -- We can finally do single cell proteomics!!!". News in Proteomics Research. 2017-03-09. Retrieved 2017-06-28.
- ↑ "Single-cell proteomics - Slavov Lab Blog". Slavov Lab Blog. 2017-06-06. Retrieved 2017-06-27.
- ↑ Wiśniewski JR, Zougman A, Nagaraj N, Mann M (May 2009). "Universal sample preparation method for proteome analysis". Nature Methods. 6 (5): 359–62. doi:10.1038/nmeth.1322. PMID 19377485.
- ↑ Smits AH, Lindeboom RG, Perino M, van Heeringen SJ, Veenstra GJ, Vermeulen M (September 2014). "Global absolute quantification reveals tight regulation of protein expression in single Xenopus eggs". Nucleic Acids Research. 42 (15): 9880–91. doi:10.1093/nar/gku661. PMC 4150773. PMID 25056316.
- ↑ Specht, Harrison; Slavov, Nikolai (2018-04-02). "Routinely quantifying single cell proteomes: A new age in quantitative biology and medicine". PeerJ Preprints. doi:10.7287/peerj.preprints.26821v1. ISSN 2167-9843. Retrieved 2018-04-03.
- 1 2 3 Zenobi R (December 2013). "Single-cell metabolomics: analytical and biological perspectives". Science. 342 (6163): 1243259. doi:10.1126/science.1243259. PMID 24311695.
- ↑ Zhang L, Foreman DP, Grant PA, Shrestha B, Moody SA, Villiers F, Kwak JM, Vertes A (October 2014). "In situ metabolic analysis of single plant cells by capillary microsampling and electrospray ionization mass spectrometry with ion mobility separation". The Analyst. 139 (20): 5079–85. doi:10.1039/C4AN01018C. PMID 25109271.