Pyrolysis
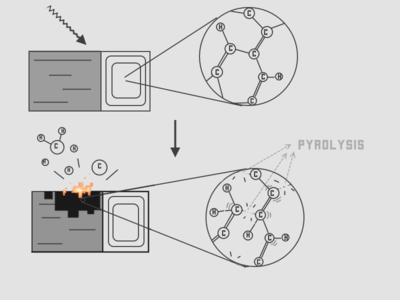
Pyrolysis is the thermal decomposition of materials at elevated temperatures in an inert atmosphere.[1] It involves the change of chemical composition and is irreversible. The word is coined from the Greek-derived elements pyro "fire" and lysis "separating".
Pyrolysis is most commonly used to the treatment of organic materials. It is one of the processes involved in charring wood, starting at 200–300 °C (390–570 °F).[2] In general, pyrolysis of organic substances produces volatile products and leaves a solid residue enriched in carbon, char. Extreme pyrolysis, which leaves mostly carbon as the residue, is called carbonization.
The process is used heavily in the chemical industry, for example, to produce ethylene, many forms of carbon, and other chemicals from petroleum, coal, and even wood, to produce coke from coal. Aspirational applications of pyrolysis would convert biomass into syngas and biochar, waste plastics back into usable oil, or waste into safely disposable substances.
Process terminology and mechanism
Special cases of pyrolysis include dry distillation, destructive distillation, cracking, and thermal depolymerization. It generally involves the breaking of chemical bonds in molecules to give smaller molecules; but may also leave residues with larger molecular mass. The phenomenon involves exceeding the decomposition temperature of the material.
Pyrolysis differs from other processes like combustion and hydrolysis in that it usually does not involve the addition of other reagents such as oxygen (O2, in combustion) or water (in hydrolysis).[3] In practice, it is often not practical to achieve completely O2- or water-free conditions, especially as pyrolysis is often conducted on complex mixtures. The term has also been applied to the decomposition of organic material in the presence of superheated water or steam (hydrous pyrolysis), for example, in the steam cracking of oil. Pyrolysis has been assumed to take place during catagenesis, the conversion of buried organic matter to fossil fuels.
Conversely, the starting material may be heated in a vacuum to decrease the boiling point of the byproducts and avoid adverse chemical reactions. An example is the process of flash vacuum pyrolysis used in organic synthesis.
Volatile pyrolysis byproducts can be analyzed through separation by gas chromatography and identification by mass spectrometry, a process known as Py-GC-MS.[4][5] Pyrolysis is also used in carbon-14 dating.
Occurrence and uses
Ethylene
Pyrolysis is used to produce ethylene, the chemical compound produced on the largest scale industrially (>110 million tons/year in 2005). In this process, hydrocarbons from petroleum are heated to around 600 °C in the presence of steam, i.e. steam cracking. The resulting ethylene is used to make antifreeze (ethylene glycol), PVC (via vinyl chloride), and many polymers, such as polyethylene and polystyrene.[6]
Coke, carbon, charcoals, and chars
Carbon and carbon-rich materials have desirable properties but are nonvolatile, even at high temperatures. Consequently, pyrolysis is used to produce many kinds of carbon; these can be used for fuel, as reagents in steelmaking (coke), and as structural materials.
High temperature pyrolysis is used on an industrial scale to convert coal into coke for metallurgy, especially steelmaking. Volatile products are often useful, including benzene and pyridine.[7] Coke can also be produced from the solid residue left from petroleum refining.

The coke-making or "coking" process consists of heating the material in "coking ovens" to very high temperatures (up to 900 °C or 1,700 °F) so that those molecules are broken down into lighter volatile substances, which leave the vessel, and a porous but hard residue that is mostly carbon and inorganic ash. The amount of volatiles varies with the source material, but is typically 25–30% of it by weight.
The original vascular structure of the wood and the pores created by escaping gases combine to produce a light and porous material. By starting with a dense wood-like material, such as nutshells or peach stones, one obtains a form of charcoal with particularly fine pores (and hence a much larger pore surface area), called activated carbon, which is used as an adsorbent for a wide range of chemical substances.
Biochar is the residue of incomplete organic pyrolysis, e.g., from cooking fires. They are a key component of the terra preta soils associated with ancient indigenous communities of the Amazon basin.[8] Terra preta is much sought by local farmers for its superior fertility compared to the natural red soil of the region. Efforts are underway to recreate these soils through biochar, the solid residue of pyrolysis of various materials, mostly organic waste.
Carbon fibers are filaments of carbon that can be used to make very strong yarns and textiles. Carbon fiber items are often produced by spinning and weaving the desired item from fibers of a suitable polymer, and then pyrolyzing the material at a high temperature (from 1,500–3,000 °C or 2,730–5,430 °F). The first carbon fibers were made from rayon, but polyacrylonitrile has become the most common starting material. For their first workable electric lamps, Joseph Wilson Swan and Thomas Edison used carbon filaments made by pyrolysis of cotton yarns and bamboo splinters, respectively.
Pyrolysis is the reaction used to coat a preformed substrate with a layer of pyrolytic carbon. This is typically done in a fluidized bed reactor heated to 1,000–2,000 °C or 1,830–3,630 °F. Pyrolytic carbon coatings are used in many applications, including artificial heart valves.[9]
Biofuels
Pyrolysis is the basis of several methods for producing fuel from biomass, i.e. lignocellulosic biomass.[10] Crops studied as biomass feedstock for pyrolysis include native North American prairie grasses such as switchgrass and bred versions of other grasses such as Miscantheus giganteus. Other sources of organic matter as feedstock for pyrolysis include greenwaste, sawdust, waste wood, nut shells, straw, cotton trash, rice hulls. Animal waste including poultry litter, dairy manure, and potentially other manures are also under evaluation. Some industrial byproducts are also suitable feedstock including paper sludge and distillers grain.[11]
Synthetic diesel fuel by pyrolysis of organic materials is not yet economically competitive.[12] Higher efficiency is sometimes achieved by flash pyrolysis, in which finely divided feedstock is quickly heated to between 350 and 500 °C (660 and 930 °F) for less than 2 seconds.
The low quality of oils produced through pyrolysis can be improved by physical and chemical processes,[13] which might drive up production costs, but may make sense economically as circumstances change.
There is also the possibility of integrating with other processes such as mechanical biological treatment and anaerobic digestion.[14] Fast pyrolysis is also investigated for biomass conversions.[15] Fuel bio-oil can also be produced by hydrous pyrolysis.
Semiconductors
The process of metalorganic vapour phase epitaxy (MOCVD) entails pyrolysis of volatile organometallic compounds to give semiconductors, hard coatings, and other applicable materials. The reactions entail thermal degradation of precursors, with deposition of the inorganic component and release of the hydrocarbons as gaseous waste. Since it is an atom-by-atom deposition, these atoms organize themselves into crystals to form the bulk semiconductor. Silicon chips are produced by the pyrolysis of silane:
- SiH4 → Si + 2 H2
Gallium arsenide, another semiconductor, forms upon co-pyrolysis of trimethylgallium and arsine.
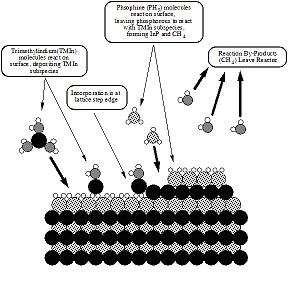
Recycling
Pyrolysis can also be used to treat plastic waste. The main advantage is the reduction in volume of the waste. In principle, pyrolysis will regenerate the monomers (precursors) to the polymers that are treated, but in practice the process is neither a clean nor an economically competitive source of monomers.[16][17][18]
In tire recycling, tire pyrolysis is well developed technology.[19] Other products from car tire pyrolysis include steel wires, carbon black and bitumen.[20] The area faces legislative, economic, and marketing obstacles.[21] Oil derived from tire rubber pyrolysis contains high sulfur content, which gives it high potential as a pollutant and should be desulfurized.[22][23]
Thermal cleaning
Pyrolysis is also used for thermal cleaning, an industrial application to remove organic substances such as polymers, plastics and coatings from parts, products or production components like extruder screws, spinnerets[24] and static mixers. During the thermal cleaning process, at temperatures between 600 °F to 1000 °F (310 C° to 540 C°),[25] organic material is converted by pyrolysis and oxidation into volatile organic compounds, hydrocarbons and carbonized gas.[26] Inorganic elements remain.[27]
Several types of thermal cleaning systems use pyrolysis:
- Molten Salt Baths belong to the oldest thermal cleaning systems; cleaning with a molten salt bath is very fast but implies the risk of dangerous splatters, or other potential hazards connected with the use of salt baths, like explosions or highly toxic hydrogen cyanide gas.[25]
- Fluidized Bed Systems[28] use sand or aluminium oxide as heating medium;[29] these systems also clean very fast but the medium does not melt or boil, nor emit any vapors or odors;[25] the cleaning process takes one to two hours.[26]
- Vacuum Ovens use pyrolysis in a vacuum[30] avoiding uncontrolled combustion inside the cleaning chamber;[25] the cleaning process takes 8[26] to 30 hours.[31]
- Burn-Off Ovens, also known as Heat-Cleaning Ovens, are gas-fired and used in the painting, coatings, electric motors and plastics industries for removing organics from heavy and large metal parts.[32]
Fine chemical synthesis
Pyrolysis is used in the production of chemical compounds, mainly, but not only, in the research laboratory.
The area of boron-hydride clusters started with the study of the pyrolysis of diborane (B2H6) at ca. 200 °C. Products include the clusters pentaborane and decaborane. These pyrolyses involve not only cracking (to give H2), but also recondensation.[33]
The synthesis of nanoparticles,[34] zirconia[35] and oxides[36] utilizing an ultrasonic nozzle in a process called ultrasonic spray pyrolysis (USP).
History
Pyrolysis has been used for turning wood into charcoal since ancient times. Many important chemical substances, such as phosphorus and sulfuric acid, were first obtained by this process. In their embalming process, the ancient Egyptians used methanol, which they obtained from the pyrolysis of wood. The dry distillation of wood remained the major source of methanol into the early 20th Century.[37]
See also
References
- ↑ "Compendium of Chemical Terminology". International Union of Pure and Applied Chemistry. 2009. p. 1824. Retrieved 2018-01-10.
- ↑ Burning of wood Archived 2010-02-09 at the Wayback Machine., InnoFireWood's website. Accessed on 2010-02-06.
- ↑ Cory A. Kramer, Reza Loloee, Indrek S. Wichman and Ruby N. Ghosh, 2009, Time Resolved Measurements of Pyrolysis Products From Thermoplastic Poly-Methyl-Methacrylate (PMMA) Archived 2014-11-06 at the Wayback Machine. ASME 2009 International Mechanical Engineering Congress and Exposition
- ↑ Goodacre, R.; Kell, D. B. (1996). "Pyrolysis mass spectrometry and its applications in biotechnology". Curr. Opin. Biotechnol. 7: 20–28. doi:10.1016/S0958-1669(96)80090-5.
- ↑ Peacock, P. M.; McEwen, C. N. (2006). "Mass Spectrometry of Synthetic Polymers. Anal. Chem". 78: 3957–3964. doi:10.1021/ac0606249.
- ↑ Zimmermann, Heinz; Walz, Roland (2008). "Ethylene". Ullmann's Encyclopedia of Industrial Chemistry. Weinheim: Wiley-VCH. doi:10.1002/14356007.a10_045.pub3.
- ↑ Ludwig Briesemeister, Andreas Geißler, Stefan Halama, Stephan Herrmann, Ulrich Kleinhans, Markus Steibel, Markus Ulbrich, Alan W. Scaroni, M. Rashid Khan, Semih Eser, Ljubisa R. Radovic (2002). "Coal Pyrolysis". Ullmann's Encyclopedia of Industrial Chemistry. Weinheim: Wiley-VCH. doi:10.1002/14356007.a07_245.pub2.
- ↑ Lehmann, Johannes. "Biochar: the new frontier". Archived from the original on 2008-06-18. Retrieved 2008-07-10.
- ↑ Ratner, Buddy D. (2004). Pyrolytic carbon. In Biomaterials science: an introduction to materials in medicine Archived 2014-06-26 at the Wayback Machine.. Academic Press. pp. 171-180. ISBN 0-12-582463-7.
- ↑ Evans, G. "Liquid Transport Biofuels – Technology Status Report" Archived September 19, 2008, at the Wayback Machine., "National Non-Food Crops Centre", 14-04-08. Retrieved on 2009-05-05.
- ↑ "Biomass Feedstock for Slow Pyrolysis". BEST Pyrolysis, Inc. website. BEST Energies, Inc. Archived from the original on 2012-01-02. Retrieved 2010-07-30.
- ↑ "Pyrolysis and Other Thermal Processing". US DOE. Archived from the original on 2007-08-14.
- ↑ Ramirez, Jerome; Brown, Richard; Rainey, Thomas (1 July 2015). "A Review of Hydrothermal Liquefaction Bio-Crude Properties and Prospects for Upgrading to Transportation Fuels". Energies. 8: 6765–6794. doi:10.3390/en8076765.
- ↑ Marshall, A. T. & Morris, J. M. (2006) A Watery Solution and Sustainable Energy Parks Archived 2007-09-28 at the Wayback Machine., CIWM Journal, pp. 22–23
- ↑ Westerhof, Roel Johannes Maria (2011). Refining fast pyrolysis of biomass. Thermo-Chemical Conversion of Biomass (Thesis). University of Twente. Archived from the original on 2013-06-17. Retrieved 2012-05-30.
- ↑ Kaminsky, Walter (2000). "Plastics, Recycling". Ullmann's Encyclopedia of Industrial Chemistry. Weinheim: Wiley-VCH. doi:10.1002/14356007.a21_057.
- ↑ N.J. Themelis et al. "Energy and Economic Value of Nonrecyclable Plastics and Municipal Solid Wastes that are Currently Landfilled in the Fifty States" Columbia University Earth Engineering Center Archived 2014-05-08 at the Wayback Machine.
- ↑ The Plastic to Oil Machine | A\J – Canada's Environmental Voice Archived 2015-09-09 at the Wayback Machine.. Alternativesjournal.ca (2016-12-07). Retrieved on 2016-12-16.
- ↑ ผศ.ดร.ศิริรัตน์ จิตการค้า, "ไพโรไลซิสยางรถยนต์หมดสภาพ : กลไกการผลิตน้ำมันเชื้อเพลิงคุณภาพสูง"วิทยาลัยปิโตรเลียมและปิโตรเคมี จุฬาลงกรณ์มหาวิทยาลัย (in Thai) Jidgarnka, S. "Pyrolysis of Expired Car Tires: Mechanics of Producing High Quality Fuels" Archived 2015-02-20 at the Wayback Machine.. Chulalongkorn University Department of Petrochemistry
- ↑ Roy, C.; Chaala, A.; Darmstadt, H. (1999). "The vacuum pyrolysis of used tires". Journal of Analytical and Applied Pyrolysis. 51: 201. doi:10.1016/S0165-2370(99)00017-0.
- ↑ Martínez, Juan Daniel; Puy, Neus; Murillo, Ramón; García, Tomás; Navarro, María Victoria; Mastral, Ana Maria (2013). "Waste tyre pyrolysis – A review, Renewable and Sustainable". Energy Reviews. 23: 179–213. doi:10.1016/j.rser.2013.02.038.
- ↑ Choi, G.-G.; Jung, S.-H.; Oh, S.-J.; Kim, J.-S. (2014). "Total utilization of waste tire rubber through pyrolysis to obtain oils and CO2 activation of pyrolysis char". Fuel Processing Technology. 123: 57. doi:10.1016/j.fuproc.2014.02.007.
- ↑ Ringer, M.; Putsche, V.; Scahill, J. (2006) Large-Scale Pyrolysis Oil Production: A Technology Assessment and Economic Analysis Archived 2016-12-30 at the Wayback Machine.; NREL/TP-510-37779; National Renewable Energy Laboratory (NREL), Golden, CO.
- ↑ Heffungs, Udo (June 2010). "Effective Spinneret Cleaning". Fiber Journal. Archived from the original on 30 June 2016. Retrieved 19 April 2016.
- 1 2 3 4 Mainord, Kenneth (September 1994). "Cleaning with Heat: Old Technology with a Bright New Future" (PDF). Pollution Prevention Regional Information Center. The Magazine of Critical Cleaning Technology. Archived (PDF) from the original on 8 December 2015. Retrieved 4 December 2015.
- 1 2 3 "A Look at Thermal Cleaning Technology". ThermalProcessing.org. Process Examiner. 14 March 2014. Archived from the original on 8 December 2015. Retrieved 4 December 2015.
- ↑ Davis, Gary; Brown, Keith (April 1996). "Cleaning Metal Parts and Tooling" (PDF). Pollution Prevention Regional Information Center. Process Heating. Archived (PDF) from the original on 4 March 2016. Retrieved 4 December 2015.
- ↑ Schwing, Ewald; Uhrner, Horst (7 October 1999). "Method for removing polymer deposits which have formed on metal or ceramic machine parts, equipment and tools". Espacenet. European Patent Office. Retrieved 19 April 2016.
- ↑ Staffin, Herbert Kenneth; Koelzer, Robert A. (28 November 1974). "Cleaning objects in hot fluidised bed – with neutralisation of resultant acidic gas esp. by alkaline metals cpds". Espacenet. European Patent Office. Retrieved 19 April 2016.
- ↑ Dwan, Thomas S. (2 September 1980). "Process for vacuum pyrolysis removal of polymers from various objects". Espacenet. European Patent Office. Retrieved 26 December 2015.
- ↑ "Vacuum pyrolysis systems". thermal-cleaning.com. Archived from the original on 15 February 2016. Retrieved 11 February 2016.
- ↑ "Paint Stripping: Reducing Waste and Hazardous Material". Minnesota Technical Assistance Program. University of Minnesota. July 2008. Archived from the original on 8 December 2015. Retrieved 4 December 2015.
- ↑ Greenwood, Norman N.; Earnshaw, Alan (1997). Chemistry of the Elements (2nd ed.). Butterworth-Heinemann. ISBN 0-08-037941-9. gives Greenwood, Norman N.; Earnshaw, Alan (1997). Chemistry of the Elements (2nd ed.). Butterworth-Heinemann. ISBN 0-08-037941-9.
- ↑ Pingali, Kalyana C.; Rockstraw, David A.; Deng, Shuguang (2005). "Silver Nanoparticles from Ultrasonic Spray Pyrolysis of Aqueous Silver Nitrate" (PDF). Aerosol Science and Technology. 39 (10): 1010–1014. doi:10.1080/02786820500380255. Archived (PDF) from the original on 2014-04-08.
- ↑ Song, Y. L.; Tsai, S. C.; Chen, C. Y.; Tseng, T. K.; Tsai, C. S.; Chen, J. W.; Yao, Y. D. (2004). "Ultrasonic Spray Pyrolysis for Synthesis of Spherical Zirconia Particles" (PDF). Journal of the American Ceramic Society. 87 (10): 1864–1871. doi:10.1111/j.1151-2916.2004.tb06332.x. Archived (PDF) from the original on 2014-04-08.
- ↑ Hamedani, Hoda Amani (2008) Investigation of Deposition Parameters in Ultrasonic Spray Pyrolysis for Fabrication of Solid Oxide Fuel Cell Cathode Archived 2016-03-05 at the Wayback Machine., Georgia Institute of Technology
- ↑ E. Fiedler, G. Grossmann, D. B. Kersebohm, G. Weiss, Claus Witte (2005). "Methanol". Ullmann's Encyclopedia of Industrial Chemistry. Weinheim: Wiley-VCH. doi:10.1002/14356007. ISBN 978-3527306732.
External links
The dictionary definition of pyrolysis at Wiktionary- In Situ Catalytic Fast Pyrolysis Technology Pathway National Renewable Energy Laboratory