Cell polarity
Cell polarity refers to spatial differences in shape, structure, and function within a cell. Almost all cell types exhibit some form of polarity, which enables them to carry out specialized functions. Classical examples of polarized cells are described below, including epithelial cells with apical-basal polarity, neurons in which signals propagate in one direction from dendrites to axons, and migrating cells. Furthermore, cell polarity is important during many types of asymmetric cell division to set up functional asymmetries between daughter cells.
Many of the key molecular players implicated in cell polarity are well conserved. For example, in metazoan cells, the PAR-3/PAR-6/aPKC complex plays a fundamental role in cell polarity. While the biochemical details may vary, some of the core principles such as negative and/or positive feedback between different molecules are common and essential to many known polarity systems.[1]
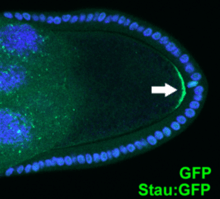
Examples of polarized cells
Epithelial cells
Epithelial cells adhere to one another through tight junctions, desmosomes and adherens junctions, forming sheets of cells that line the surface of the animal body and internal cavities (e.g., digestive tract and circulatory system). These cells have an apical-basal polarity defined by the apical membrane facing the outside surface of the body, or the lumen of internal cavities, and the basolateral membrane oriented away from the lumen. The basolateral membrane refers to both the lateral membrane where cell-cell junctions connect neighboring cells and to the basal membrane where cells are attached to the basement membrane, a thin sheet of extracellular matrix proteins that separates the epithelial sheet from underlying cells and connective tissue. Epithelial cells also exhibit planar cell polarity,in which specialized structures are orientated within the plane of the epithelial sheet. Some examples of planar cell polarity include the scales of fish being oriented in the same direction and similarly the feathers of birds, the fur of mammals, and the cuticular projections (sensory hairs, etc.) on the bodies and appendages of flies and other insects.[2]
Neurons
A neuron receives signals from neighboring cells through branched, cellular extensions called dendrites. The neuron then propagates an electrical signal down a specialized axon extension to the synapse, where neurotransmitters are released to propagate the signal to another neuron or effector cell (e.g., muscle or gland). The polarity of the neuron thus facilitates the directional flow of information, which is required for communication between neurons and effector cells.[3]
Migratory cells
Many cell types are capable of migration, such as leukocytes and fibroblasts, and in order for these cells to move in one direction, they must have a defined front and rear. At the front of the cell is the leading edge, which is often defined by a flat ruffling of the cell membrane called the lamellipodium or thin protrusions called filopodia. Here, actin polymerization in the direction of migration allows cells to extend the leading edge of the cell and to attach to the surface.[4] At the rear of the cell, adhesions are disassembled and bundles of actin microfilaments, called stress fibers, contract and pull the trailing edge forward to keep up with the rest of the cell. Without this front-rear polarity, cells would be unable to coordinate directed migration.[5]
Budding yeast
Main article: Polarity establishment in yeast
Generation of cellular polarity is critically important for the function of practically every cell type and underlies processes like cell division, differentiation, cell migration, cell–cell signalling and fertilization. Cell polarity is an example of the self-organization property that all living organism share. All the cells within a multicellular organism, or any single cell species i.e. yeast, displays a polarized organization necessary for its proliferation, differentiation or physiological function. Budding yeast is a highly accessible experimental system, which serves as a paradigm for deciphering the molecular mechanism underlying the generation of polarity. Yeast cells share many features about cell polarity with other organism like: regulation by intrinsic and extrinsic cues, conserved regulatory molecules such as Cdc42GTPase, and asymmetry of the cytoskeleton. Cell polarization is associated with a type of symmetry breaking, that occurs in the cytoskeleton and guides the direction of growth of the future daughter cell. This symmetry breaking facilitates the polarized flux and localization of several protein in the polarized patch. When cells can perform symmetry breaking in absence of any spatial cue (landmarks),[6] is called spontaneous polarization or spontaneous symmetry breaking.
In short, polarity establishment or symmetry breaking, in this context, is the first step for cell polarity and consequently cell division. We are interested in the spontaneous symmetry breaking, as an example of self-organization phenomena in living cells. One of the way, and one of the most popular ones, to study spontaneous symmetry breaking is by correlating it with a type of reaction- diffusion phenomena.
The molecular identity of most important proteins involved in leading spontaneous symmetry breaking have been extensively studied.[7] Those proteins have been categorized in general modules that represent the main functional cores for yeast life cycle.[8] However, the molecular mechanisms responsible for this regulatory network are still poorly understood. Extensive work done in many organisms ranging from prokaryotes to high complex ones has revealed a central role of small GTPases in the cell polarization process. Particularly in yeast, this protein is Cdc42, which is a member of the eukaryotic Ras-homologous Rho-family of GTPases, which is part of the wider super-family of small GTPases, including Rop GTPases in plants and small GTPases in prokaryotes.
A recent study to elucidate the connection between cell cycle timing and Cdc42 accumulation in the bud site uses optogenetics to control protein localization using light.[9]
Furthermore, using experimental evolution, function and robustness of yeast polarity establishment have been studied.[10]
Vertebrate development
The bodies of vertebrate animals are asymmetric along three axes: anterior-posterior (head to tail), dorsal-ventral (spine to belly), and left-right (for example, our heart is on the left side of our body). These polarities arise within the developing embryo through a combination of several processes: 1) asymmetric cell division, in which two daughter cells receive different amounts of cellular material (e.g. mRNA, proteins), 2) asymmetric localization of specific proteins or RNAs within cells (which is often mediated by the cytoskeleton), 3) concentration gradients of secreted proteins across the embryo such as Wnt, Nodal, and Bone Morphogenic Proteins (BMPs), and 4) differential expression of membrane receptors and ligands that cause lateral inhibition, in which the receptor-expressing cell adopts one fate and its neighbors another.[11][12]
In addition to defining asymmetric axes in the adult organism, cell polarity also regulates both individual and collective cell movements during embryonic development such as apical constriction, invagination, and epiboly. These movements are critical for shaping the embryo and creating the complex structures of the adult body.
Molecular basis
Cell polarity arises primarily through the localization of specific proteins to specific areas of the cell membrane. This localization often requires both the recruitment of cytoplasmic proteins to the cell membrane and polarized vesicle transport along cytoskeletal filaments to deliver transmembrane proteins from the golgi apparatus. Many of the molecules responsible for regulating cell polarity are conserved across cell types and throughout metazoan species. Examples include the PAR complex (Cdc42, PAR3/ASIP, PAR6, atypical protein kinase C),[13][14] Crumbs complex (Crb, PALS, PATJ, Lin7), and Scribble complex (Scrib, Dlg, Lgl).[15] These polarity complexes are localized at the cytoplasmic side of the cell membrane, asymmetrically within cells. For example, in epithelial cells the PAR and Crumbs complexes are localized along the apical membrane and the Scribble complex along the lateral membrane.[16] Together with a group of signaling molecules called Rho GTPases, these polarity complexes can regulate vesicle transport and also control the localization of cytoplasmic proteins primarily by regulating the phosphorylation of phospholipids called phosphoinositides. Phosphoinositides serve as docking sites for proteins at the cell membrane, and their state of phosphorylation determines which proteins can bind.[17]
Polarity establishment
While many of the key polarity proteins are well conserved, different mechanisms exist to establish cell polarity in different cell types. Here, two main classes can be distinguished: cells that are able to polarize spontaneously and cells that establish polarity based on intrinsic or environmental cues.[18]
Spontaneous symmetry breaking can be explained by amplification of stochastic fluctuations of molecules due to non-linear chemical kinetics. The mathematical basis for this biological phenomenon was established by Alan Turing in his 1953 paper 'The chemical basis of morphogensis'.[19] While Turing initially attempted to explain pattern formation in a multicellular system, similar mechanisms can also be applied to intracellular pattern formation.[20] Briefly, if a network of at least two interacting chemicals (in this case proteins) exhibits certain types of reaction kinetics, as well as differential diffusion, stochastic concentration fluctuations can give rise to the formation of large-scale stable patterns, thus, bridging from a molecular length scale to a cellular or even tissue scale.
A prime example for the second type of polarity establishment, which relies on extracellular or intracellular cues, is the C. elegans zygote. Here, mutual inhibition between two sets of proteins guides polarity establishment and maintenance. On the one hand PAR-3, PAR-6 and aPKC (called anterior PAR proteins) occupy both plasma membrane and the cytoplasm prior to symmetry breaking. PAR-1, the C. elegans specific ring-finger-containing protein PAR-2, and LGL-1 (called posterior PAR proteins) are present mostly in the cytoplasm.[21] The male centrosome provides a cue, which breaks an initially homogenous membrane distribution of anterior PARs by inducing cortical flows. These are thought to advect anterior PARs towards one side of the cell, allowing posterior PARs to bind to other pole (posterior).[22][23] Anterior and posterior PAR proteins then maintain polarity until cytokinesis by mutually excluding each other from their respective cell membrane areas.
See also
References
- ↑ Altschuler, Steven J.; Angenent, Sigurd B.; Wang, Yanqin; Wu, Lani F. (August 2008). "On the spontaneous emergence of cell polarity". Nature. 454 (7206): 886. doi:10.1038/nature07119. ISSN 1476-4687. PMC 2562338.
- ↑ Wu, Jun; Mlodzik, Marek A. (29 June 2009). "A quest for the mechanism regulating global planar cell polarity of tissues". Trends in Cell Biology. 19 (7): 295–305. doi:10.1016/j.tcb.2009.04.003. PMC 3501338. PMID 19560358.
- ↑ Rasband, Matthew N. (August 2010). "The axon initial segment and the maintenance of neuronal polarity". Nature Reviews Neuroscience. 11 (8): 552–562. doi:10.1038/nrn2852.
- ↑ Ridley, Anne J.; Schwartz, Martin A.; Burridge, Keith; Firtel, Richard A.; Ginsberg, Mark H.; Borisy, Gary; Parsons, J. Thomas; Horwitz, Alan Rick (5 December 2003). "Cell Migration: Integrating Signals from Front to back". Science. 302 (5651): 1704–1709. doi:10.1126/science.1092053. PMID 14657486.
- ↑ Friedl, Peter; Wolf, Katarina (May 2003). "Tumour-cell invasion and migration: diversity and escape mechanisms". Nature Reviews Cancer. 3 (5): 362–374. doi:10.1038/nrc1075. PMID 12724734.
- ↑ Wedlich-Soldner, Roland; Li, Rong (2003-04-01). "Spontaneous cell polarization: undermining determinism". Nature Cell Biology. 5 (4): 267–270. doi:10.1038/ncb0403-267.
- ↑ Bi, Erfei; Park, Hay-Oak (June 2012). "Cell polarization and cytokinesis in budding yeast". Genetics. 191 (2): 347–387. doi:10.1534/genetics.111.132886. ISSN 1943-2631. PMC 3374305. PMID 22701052.
- ↑ Irazoqui, Javier E.; Lew, Daniel J. (2004-05-01). "Polarity establishment in yeast". Journal of Cell Science. 117 (11): 2169–2171. doi:10.1242/jcs.00953. ISSN 0021-9533. PMID 15126618.
- ↑ Witte, Kristen; Strickland, Devin; Glotzer, Michael (2017-07-06). "Cell cycle entry triggers a switch between two modes of Cdc42 activation during yeast polarization". eLife. 6. doi:10.7554/eLife.26722. ISSN 2050-084X.
- ↑ Laan, Liedewij; Koschwanez, John H; Murray, Andrew W (2015-10-01). "Evolutionary adaptation after crippling cell polarization follows reproducible trajectories". eLife. 4. doi:10.7554/eLife.09638. ISSN 2050-084X.
- ↑ Hashimoto, Masakazu; Hamada, Hiroshi (August 2010). "Translation of anterior-posterior polarity into left-right polarity in the mouse embryo". Current Opinion in Genetics & Development. 20 (4): 433–437. doi:10.1016/j.gde.2010.04.002. PMID 20439159.
- ↑ Johnston, Daniel St; Ahringer, Julie (28 May 2010). "Cell Polarity in Eggs and Epithelia: Parallels and Diversity". Cell. 141 (5): 757–774. doi:10.1016/j.cell.2010.05.011. PMID 20510924.
- ↑ Izumi Y, Hirose T, Tamai Y, Hirai S, Nagashima Y, Fujimoto T, Tabuse Y, Kemphues KJ, Ohno S (October 1998). "An Atypical PKC Directly Associates and Colocalizes at the Epithelial Tight Junction with ASIP, a Mammalian Homologue of Caenorhabditis elegans Polarity Protein PAR-3". J Cell Biol. 143 (1): 95–106. doi:10.1083/jcb.143.1.95. PMC 2132825. PMID 9763423.
- ↑ Tabuse Y, Izumi Y, Piano F, Kemphues KJ, Miwa J, Ohno S (September 1998). "Atypical protein kinase C cooperates with PAR-3 to establish embryonic polarity in Caenorhabditis elegans". Development. 125 (18): 3607–3614. PMID 9716526.
- ↑ Bryant, David M.; Mostov, Keith E. (November 2008). "From cells to organs: building polarized tissue". Nature Reviews Molecular Cell Biology. 9 (11): 887–901. doi:10.1038/nrm2523. PMC 2921794.
- ↑ Johnston, Daniel St; Ahringer, Julie (28 May 2010). "Cell Polarity in Eggs and Epithelia: Parallels and Diversity". Cell. 141 (5): 757–774. doi:10.1016/j.cell.2010.05.011. PMID 20510924.
- ↑ Orlando, Kelly; Guo, Wei (November 2009). "Organization and Dynamics in Cell Polarity". Cold Spring Harbor Perspectives in Biology. 1 (5): a001321. doi:10.1101/cshperspect.a001321. PMC 2773647.
- ↑ Wedlich-Soldner, Roland; Li, Rong (2003-04-01). "Spontaneous cell polarization: undermining determinism". Nature Cell Biology. 5 (4): 267–270. doi:10.1038/ncb0403-267.
- ↑ Turing, A. M.; S, F. R. (1952-08-14). "The chemical basis of morphogenesis". Phil. Trans. R. Soc. Lond. B. 237 (641): 37–72. doi:10.1098/rstb.1952.0012. ISSN 0080-4622.
- ↑ Gierer, A.; Meinhardt, H. (1972-12-01). "A theory of biological pattern formation". Kybernetik. 12 (1): 30–39. doi:10.1007/BF00289234. ISSN 0023-5946.
- ↑ "Asymmetric cell division and axis formation in the embryo". www.wormbook.org. Retrieved 2018-04-06.
- ↑ Munro, Edwin; Nance, Jeremy; Priess, James R. (2004-09-01). "Cortical Flows Powered by Asymmetrical Contraction Transport PAR Proteins to Establish and Maintain Anterior-Posterior Polarity in the Early C. elegans Embryo". Developmental Cell. 7 (3): 413–424. doi:10.1016/j.devcel.2004.08.001. ISSN 1534-5807.
- ↑ Goehring, Nathan W.; Trong, Philipp Khuc; Bois, Justin S.; Chowdhury, Debanjan; Nicola, Ernesto M.; Hyman, Anthony A.; Grill, Stephan W. (2011-11-25). "Polarization of PAR Proteins by Advective Triggering of a Pattern-Forming System". Science. 334 (6059): 1137–1141. doi:10.1126/science.1208619. ISSN 0036-8075. PMID 22021673.