Serpentine soil
Serpentine soil is an uncommon soil type produced by weathered ultramafic rock such as peridotite and its metamorphic derivatives such as serpentinite. More precisely, serpentine soil contains minerals of the serpentine subgroup, especially antigorite, lizardite, and chrysotile or white asbestos, all of which are commonly found in ultramafic rocks. The term "serpentine" is commonly used to refer to both the soil type and the mineral group which forms its parent materials.

Serpentine soils exhibit distinct chemical and physical properties and are generally regarded as poor soils. The soil is often reddish, brown, or gray in color due to its high iron and low organic content. Geologically, areas with serpentine bedrock are characteristically steep, rocky, and vulnerable to erosion, which causes many serpentine soils to be rather shallow.[1] The shallow soils and sparse vegetation lead to elevated soil temperatures[2] and dry conditions.[1] Due to their ultramafic origin, serpentine soils also suffer from a low calcium-to-magnesium ratio and lack many essential nutrients such as nitrogen (N), phosphorus (P), and potassium (K). Serpentine soils contain high concentrations of heavy metals, including chromium, iron, cobalt, and nickel.[3] Together, these factors create serious ecological challenges for plants living in serpentine soils.
Parent rock
Serpentinite is a meta-igneous rock formed by the metamorphic reaction of olivine-rich rock, peridotite, with water. Serpentinite has a mottled, greenish-gray or bluish-gray color and is often waxy to the touch. The rock often contains white streaks of chrysotile running through it, which are a type of naturally occurring asbestos. Asbestos is linked to an array of human health conditions such as mesothelioma from long time exposure of breathing in the dust particles. Caution should be taken when working in serpentine soils or when working with crushed serpentine rocks.
Serpentinite most often forms in oceanic crust near the surface of the earth, particularly where water circulates in cooling rock near mid-ocean ridges: masses of the resulting ultramafic rock are found in ophiolites incorporated in continental crust near present and past tectonic plate boundaries.
Serpentine soils are derived from ultramafic rocks. Ultramafic rocks are igneous or metamorphic rocks that contain more than 70% iron or magnesium. [4]
Distribution

Serpentine soils are widely distributed on Earth, in part mirroring the distribution of ophiolites. There are outcroppings of serpentine soils in the Balkan Peninsula, Turkey, Alps, Cuba, and New Caledonia.[2] In North America, serpentine soils also are present in small but widely distributed areas on the eastern slope of the Appalachian mountains in the eastern United States. [5] However, California has the majority of the continent's serpentine soils.
Botany
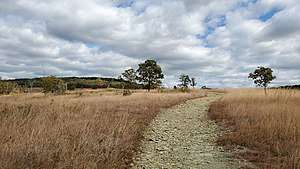
Ecologically, serpentine soils have three main traits: poor plant productivity, high rates of endemism, and vegetation types which are distinct from neighboring areas.[6]
Serpentine plant communities range from moist bogs and fens to rocky barrens, and must be able to tolerate the harsh environmental conditions of such poor soil. As a result, they are often drastically different than non-serpentine soil areas bordering the serpentine soils.[4] Vegetative characteristics are often shared among the types of flora found on serpentine soils. They will exhibit a "stunted" growth habit, with dull waxy, gray-green leaves (seen in Eriogonum libertini), which allow for water retention and sunlight reflection respectively.[7] Other possible phenotypic traits include pigmented stems (as seen in the Streptanthus howellii) and occasionally a carnivorous nature as seen in the Darlingtonia californica. Some examples of common serpentine tolerant plants include: Gray Pine (Pinus sabiniana), California Lilac (Ceanothus sp.), Manzanita (Arctostaphylos sp.), Live-Oak (Quercus sp.), California Redbud (Cercis occidentalis), California Buckeye (Aesculus californica), and California laurel (Bay tree) (Umbellularia californica). Areas of serpentine soil are also home to diverse plants, many of which are rare or endangered species such as Acanthomintha duttonii, Pentachaeta bellidiflora, and Phlox hirsuta. In California, 45% of the taxa associated with serpentine are rare or endangered.[8] In California, shrubs such as leather oak (Quercus durata) and coast whiteleaf manzanita (Arctostaphylos viscida ssp. pulchella) are typical of serpentine soils.[4]
In order to overcome the chemical and physical challenges presented by serpentine soils, plants have developed tolerances to drought, heavy metals, and limited nutrients.[4] Low calcium:magnesium ratios cause limited root growth and root activity, weak cell membranes, and reduced uptake of essential nutrients.[9] An adaptive mechanism to high magnesium soils allocates more resources to deep-growing roots.[7] Heavy metals stunt growth, induce iron deficiency, cause chlorosis, and restrict root development.[9][7] Multiple adaptive mechanisms to heavy metals include the exclusion of metals by restricting the uptake by the roots, compartmentalization of metals in various organs, or the development of toxicity tolerance.[7] In nitrogen-poor sites, physiological effects on plants include impaired protein synthesis, chlorosis, reduced leaf turgor, reduced leaf and tiller number, reduced growth rate, and low seed yield.[9] Low phosphorus levels cause similar effects of low nitrogen but also cause reduced seed size, lower root to shoot ratios, and increased water stress.[9] Low soil moisture causes reduced nutrient uptake and transport, decreased stomatal opening and reduced photosynthetic capacity, and also reduces plant growth and productivity.[9] Serpentine plants have strongly developed root systems to facilitate uptake of water and nutrients.[7] For example, Noccaea fendleri (aka Fendler's penny grass) is a hyper-accumulator of nickel and Sedum laxum ssp. expresses succulence. In some cases, symbioses with serpentine tolerant ectomycorrhizal help facilitate plants’ adaptation to edaphic stressors on serpentine.[7]
Adaptation to serpentine soils has evolved multiple times.[7][4][1][10] Serpentine tolerant plants are evolutionary younger than non-serpentine plants.[11] The heterogeneity of serpentine communities coupled with their patchy distribution limits gene flow but promotes speciation and diversification.[11] Habitat heterogeneity is an important contributor to the level of endemism and biodiversity in this system. Although the patchy distribution is attributed to the high rates of speciation in serpentine communities, there are a number of challenges associated with this. The spatial isolation from source and other populations limit gene flow,[4] which could make these populations vulnerable to changing environmental conditions. In addition, there is high gene flow with the non-serpentine communities that can cause genotypic pollution, hybridization and nonviable offspring.[4]
Bioremediation
The unique plants that survive in serpentine soils have been used in the process of phytoremediation, a type of bioremediation. Since these plants developed specialized adaptations to high concentrations of heavy metals, they have been used to remove heavy metals from polluted soil.[12]
Serpentine barrens
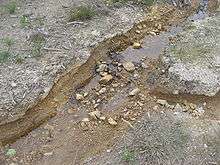
Serpentine barrens are a unique ecoregion found in parts of the United States in small but widely distributed areas of the Appalachian Mountains and the Coast Ranges of California, Oregon, and Washington.[13] Species-rich archipelagos of communities comprise 1.5% of the state's land area. In California, 10% of the state's plants are serpentine endemics. The barrens occur on outcrops of altered ultramafic ophiolites.
They are named for minerals of the serpentine group, resulting in serpentine soils, with unusually high concentrations of iron, chromium, nickel and cobalt. Serpentine barrens often consist of grassland or savannas in areas where the climate would normally lead to the growth of forests.[14]
Serpentine soils can be amended to support crops and pasture land for cattle grazing. This can be done by adding ample amounts of gypsum to the soil. By adding gypsum the soil pH (potential hydrogen) can be potentially leveled, creating an environment where more plant nutrients are in their available state. This however poses a possible implication to grazing cattle. An article from the “Journal of Trace Elements in Medicine and Biology” discerned that 20% of the grazing animals had toxic levels of nickel in their kidneys, and 32% had toxic levels of copper in their liver (Miranda et al. 2009). Further study is needed to see if this will potentially have a negative effect on human health as it pertains to beef consumption.
Examples
Soldiers Delight Natural Environmental Area in Baltimore County, Maryland, covers 1,900 acres of serpentine barren. The area has over 38 rare, threatened and endangered plant species; as well as rare insects, rocks and minerals.[15]
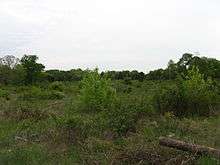
Rock Springs Nature Preserve in Lancaster County, Pennsylvania, is a 176-acre (71 ha) property conserved by the Lancaster County Conservancy that is a prime example of a serpentine barren. It was originally a grassland, but fire suppression led to the conversion of the area to forest. This barren contains the rare serpentine aster, as well as a number of rare species of moths and skippers.[16]
In Chester County, Pennsylvania, the Nottingham Park, aka Serpentine Barrens, was recommended by UMCES as deserving of National Natural Landmark designation, on numerous grounds. They included supporting a number of rare and endemic species, an intact population of pitch pine, and also the site having historic significance.[14]
See also
- Pygmy forest
References
- Brady, Kristy U.; Kruckeberg, Arthur R.; Bradshaw Jr., H.D. (2005). "Evolutionary Ecology of Plant Adaptation to Serpentine Soils". Annual Review of Ecology, Evolution, and Systematics. 36: 243–266. doi:10.1146/annurev.ecolsys.35.021103.105730.
- Kruckeberg, Arthur R (2002). Geology and plant life : the effects of landforms and rock types on plants. University of Washington Press. ISBN 978-0-295-98203-8. OCLC 475373672.
- Chiarucci, Alessandro; Baker, Alan J. M. (2007). "Advances in the ecology of serpentine soils". Plant and Soil. 293 (1–2): 1–2. doi:10.1007/s11104-007-9268-7.
- Kruckeberg, Arthur R (2006). Introduction to California soils and plants : serpentine, vernal pools, and other geobotanical wonders. University of California Press. ISBN 978-0-520-23372-0. OCLC 928683002.
- Dann, Kevin T. (1988). Traces on the Appalachians: A Natural History of Serpentine in Eastern North America. New Brunswick: Rutgers University Press. ISBN 0-8135-1323-5.
- Whittaker, R.H. (1954). "The ecology of serpentine soils". Ecology. 35 (2): 258–288. doi:10.2307/1931126. JSTOR 1931126.
- Harrison, Susan; Rajakaruna, Nishanta (2011). Serpentine : the evolution and ecology of a model system. University of California Press. ISBN 9780520268357. OCLC 632224033.
- Safford, H. D.; Viers, J. H.; Harrison, S. P. (2005). "Serpentine Endemism in the California Flora: A Database of Serpentine Affinity". Madroño. 52 (4): 222. doi:10.3120/0024-9637(2005)52[222:SEITCF]2.0.CO;2.
- Zefferman, Emily; Stevens, Jens T.; Charles, Grace K.; Dunbar-Irwin, Mila; Emam, Taraneh; Fick, Stephen; Morales, Laura V.; Wolf, Kristina M.; Young, Derek J. N.; Young, Truman P. (2015). "Plant communities in harsh sites are less invaded: A summary of observations and proposed explanations". AoB Plants. 7: plv056. doi:10.1093/aobpla/plv056. PMC 4497477. PMID 26002746.
- Arnold, Brian J.; Lahner, Brett; Dacosta, Jeffrey M.; Weisman, Caroline M.; Hollister, Jesse D.; Salt, David E.; Bomblies, Kirsten; Yant, Levi (2016). "Borrowed alleles and convergence in serpentine adaptation" (PDF). Proceedings of the National Academy of Sciences. 113 (29): 8320–5. doi:10.1073/pnas.1600405113. PMC 4961121. PMID 27357660.
- Anacker, Brian L.; Whittall, Justen B.; Goldberg, Emma E.; Harrison, Susan P. (2011). "Origins and Consequences of Serpentine Endemism in the California Flora". Evolution. 65 (2): 365–76. doi:10.1111/j.1558-5646.2010.01114.x. PMID 20812977.
- NATO Advanced Study Institute on Phytoremediation of Metal-Contaminated Soils, Morel, J.-L., Echevarria, G., & Goncharova, N. (2006). Phytoremediation of metal-contaminated soils. NATO science series, v. 68. Dordrecht: Springer.
- Anderson, Roger C., et al., Savannas, Barrens, and Rock Outcrop Plant Communities of North America, Ch. 19, Cambridge University Press, 1999, ISBN 0-521-57322-X
- "Evaluation of the Nottingham Park Serpentine Barrens", UMCES-AL, Retrieved May 10, 2009.
- "Archived copy". Archived from the original on 2012-11-08. Retrieved 2012-11-07.CS1 maint: archived copy as title (link)
- "Rock Springs Nature Preserve", Lancaster County Conservancy Website, Retrieved May 10, 2009. Archived February 22, 2009, at the Wayback Machine
- Whittaker, R. H., Walker, R., Kruckeberg, A., ”The Ecology of Serpentine Soils”.Department of xxxRadiological Services.1954.pp 258–275.Web.http://www.californiachaparral.com/images xxx/Whittaker_Eco_of_Serpentine_Soils_1954_II.pdf.Accessed 05/2017.
- Department of Natural Resources.”Natural Communities-Serpentine grasslands”.Maryland.gov. xxxWeb. http://dnr2.maryland.gov/wildlife/Pages/plants_wildlife/serpentine.aspx.Accessed xxx05/2017.
- Miranda, M.; Benedito, J.L.; Blanco-Penedo, I.; López-Lamas, C.; Merino, A.; López-Alonso, M. (2009). "Metal accumulation in cattle raised in a serpentine-soil area: Relationship between metal concentrations in soil, forage and animal tissues". Journal of Trace Elements in Medicine and Biology. 23 (3): 231–8. doi:10.1016/j.jtemb.2009.03.004. PMID 19486833.
- Ellis, M.”Should We be Worried about Asbestos in Serpentine Rock?”. Bay Nature.2013.Web. https://baynature.org/article/should-we-be-worried-about-asbestos-in-serpentine-rock/. Accessed 05/2017.
- University of California.”Trees and Shrubs for Northern California Serpentine xxxLandscapes”.Division of Agriculture and Natural resources.2009.Web.http://anrcatalog. xxxucanr.edu/pdf/8400.pdf.Accessed 05/2017.
- USDA.”Serpentine Soils and Plant Adaptations”. United States Department of Agriculture.Web. https://www.fs.fed.us/wildflowers/beauty/serpentines/adaptations.shtml Accessed 05/2017.