History of aluminium
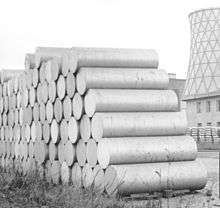
Aluminium or aluminum is a chemical element with symbol Al and atomic number 13. At standard conditions, aluminium forms a bright silvery metal; this metal is unusually light and resistant against corrosion. Chemically, aluminium is a post-transition metal that normally assumes the +3 oxidation state. Aluminium is the third most abundant element in the Earth's crust;[1] as such, it is widespread in human-related activities. Aluminium is produced in tens of millions of metric tons; the metal is commonly alloyed to improve some characteristics, such as hardness. Aluminium has no biological role but is not particularly toxic.[2]
The aluminium compound alum has been known since the 5th century BCE and was extensively used by ancients for dyeing and city defense; during the Middle Ages, the former use made alum a subject of international commerce. Scientists of the Renaissance believed alum was a salt of a new earth; during the Age of Enlightenment, it was established that the earth was an oxide of a new metal. Discovery of this metal was announced in 1825 by Danish physicist Hans Christian Ørsted, whose work was extended by German chemist Friedrich Wöhler.
Aluminium was difficult to refine and thus uncommon in actual usage. Soon after its discovery, the price of aluminium exceeded that of gold and was only reduced after the initiation of the first industrial production by French chemist Henri Étienne Sainte-Claire Deville in 1856. Aluminium became much more available to the general public with the Hall–Héroult process independently developed by French engineer Paul Héroult and American engineer Charles Martin Hall in 1886 and the Bayer process developed by Austrian chemist Carl Joseph Bayer in 1889. These processes have been used for aluminium production up to the present.
Introduction of these methods to mass production of aluminium led to the extensive use of the metal in industry and everyday lives. Aluminium has been used in aviation, engineering, construction, and packaging. Its production grew exponentially in the 20th century and it became an exchange commodity in the 1970s. In 1900, production was 6,800 metric tons; in 2015, it was 57,500,000 tons.
Early history
The history of aluminium was shaped by the usage of its compound alum. The first written record of alum was made in the 5th century BCE by Greek historian Herodotus.[3] Ancients used alum as dyeing mordants, in medicine, as a fire-resistant coating for wood, and in chemical milling.[4] Aluminium metal was unknown. Roman historian Pliny the Elder recorded a story about a metal bright as silver but much lighter. The metal was presented to the Emperor Tiberius (reigned 14–37 CE), who had the discoverer killed so the metal would not diminish the value of his gold and silver assets.[lower-alpha 1] Some sources suggest this metal could be aluminium,[lower-alpha 2] but this has been disputed.[7] It is possible that the Chinese produced aluminium-containing alloys during the reign of the first Jin dynasty (265–420).[lower-alpha 3]
After the Crusades, alum was a subject of international commerce;[10] it was indispensable in the European fabric industry.[11] Alum was imported to Europe from the eastern Mediterranean until the mid-15th century, when the Ottomans greatly raised export taxes. Small alum mines were worked in Catholic Europe. In 1460, Giovanni da Castro, godson of the Pope Pius II, discovered a rich source of alum at Tolfa near Rome and reported excitedly to his godfather, "today I bring you victory over the Turk".[lower-alpha 4]
Establishing the nature of alum
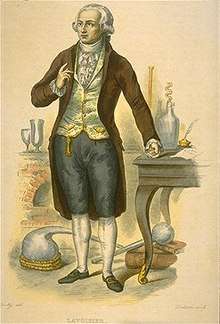
At the start of the Renaissance, the nature of alum remained unknown. Around 1530, Swiss physician Paracelsus recognized alum as separate from vitriole (sulfates) and suggested that it was a salt of an earth.[13] In 1595, German doctor and chemist Andreas Libavius demonstrated alum and green and blue vitriole were formed by the same acid but different earths;[14] for the undiscovered earth that formed alum, he proposed the name "alumina".[13] In 1702, German chemist Georg Ernst Stahl stated the unknown base of alum was akin to lime or chalk; this mistaken view was shared by many scientists for half a century.[15] In 1722, German chemist Friedrich Hoffmann suggested the base of alum was a distinct earth.[15] In 1728, French chemist Étienne Geoffroy Saint-Hilaire claimed alum was formed by an unknown earth and sulfuric acid;[15] he mistakenly believed burning of that earth yielded silica.[16] In 1739, French chemist Jean Gello proved the earth in clay and the earth resulting from the reaction of an alkali on alum were identical.[17] In 1746, German chemist Johann Heinrich Pott showed the precipitate obtained from pouring an alkali into a solution of alum was different from lime and chalk.[18]
In 1754, German chemist Andreas Sigismund Marggraf synthesized the earth of alum by boiling clay in sulfuric acid and adding potash.[15] He realized that adding soda, potash, or an alkali to a solution of the new earth in sulfuric acid yielded alum.[19] He described the earth as alkaline, as he had discovered it dissolved in acids when dried. Marggraf also described salts of this earth: the chloride, the nitrate, and the acetate.[17] In 1758, French chemist Pierre Macquer wrote that alumina[lower-alpha 5] resembled a metallic earth.[20] In 1767, Swedish chemist Torbern Bergman synthesized alum by boiling alunite in sulfuric acid and adding potash to the solution. He also synthesized alum as a reaction product between sulfates of potassium and earth of alum, demonstrating that alum was a double salt.[13] In 1776, German pharmaceutical chemist Carl Wilhelm Scheele demonstrated that both alum and silica originated from clay and alum did not contain silicon.[21] Geoffroy's mistake was only corrected in 1785 by German chemist and pharmacist Johann Christian Wiegleb who determined the earth of alum could not be synthesized from silica and alkalis, contrary to contemporary belief.[22]
Swedish chemist Jöns Jacob Berzelius suggested in 1815[23] the formula AlO3 for alumina.[24] The correct formula, Al2O3, was established by German chemist Eilhard Mitscherlich in 1821; this helped Berzelius determine the correct atomic weight of the metal, 27.[24]
Synthesis of metal
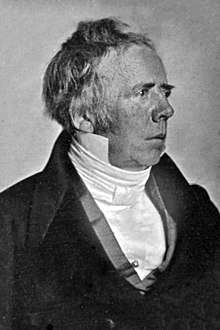
In 1760, French chemist Theodor Baron de Henouville suggested alumina was a metallic earth and attempted to reduce it to its metal, but with no success. He claimed he had tried every method of reduction known at the time, though his methods were not published. It is probable that he mixed alum with carbon or some organic substance, with salt or soda for flux, and heated it in a charcoal fire.[20] In 1782, French chemist Antoine Lavoisier wrote he considered alumina was an oxide of a metal with an affinity for oxygen so strong that no known reducing agents could overcome it.[25]
In 1790, Austrian chemists Anton Leopold Ruprecht and Matteo Tondi repeated Baron's experiments, significantly increasing the temperatures. They found small metallic particles they believed were the sought-after metal; but later experiments by other chemists showed these were iron phosphide from impurities in charcoal and bone ash. German chemist Martin Heinrich Klaproth commented in an aftermath, "if there exists an earth which has been put in conditions where its metallic nature should be disclosed, if it had such, an earth exposed to experiments suitable for reducing it, tested in the hottest fires by all sorts of methods, on a large as well as on a small scale, that earth is certainly alumina, yet no one has yet perceived its metallization."[26] Lavoisier in 1794[27] and French chemist Louis-Bernard Guyton de Morveau in 1795 melted alumina to a white enamel in a charcoal fire fed by pure oxygen but found no metal.[27] American chemist Robert Hare in 1802 melted alumina with an oxyhydrogen blowpipe, also obtaining the enamel, but still found no metal.[26]
In 1807, British chemist Humphry Davy successfully electrolyzed alumina with alkaline batteries, but the resulting alloy contained potassium and sodium, and Davy had no means to separate the desired metal from these. He then heated alumina with potassium, forming potassium oxide, but was unable to produce the sought-after metal.[26] In 1808, Davy set up a different experiment on electrolysis of alumina, establishing that alumina decomposed in the electric arc, but formed metal alloyed with iron and he was unable to separate the two.[28] Finally he tried yet another electrolysis experiment, seeking to collect the metal on iron, but was again unable to separate the coveted metal from it.[26] Davy suggested the metal be named alumium in 1808[29] and aluminum in 1812, thus producing the modern name.[28] Other scientists used the spelling aluminium; the former spelling regained usage in the United States in the following decades.[30]
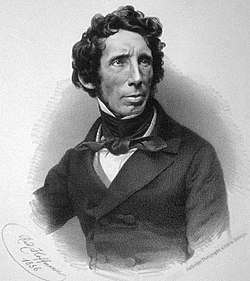
In 1813, American chemist Benjamin Silliman repeated Hare's experiment and obtained small granules of the sought-after metal, which almost immediately burned.[26]
Production of the metal was claimed in 1824 by Danish physicist and chemist Hans Christian Ørsted. He reacted anhydrous aluminium chloride with potassium amalgam, yielding a lump of metal that looked similar to tin.[31][32] He presented his results and demonstrated a sample of the new metal in 1825. In 1826, he wrote, "aluminium has a metallic luster and somewhat grayish color and breaks down water very slowly"; this suggests that he had obtained an aluminium–potassium alloy rather than pure aluminium.[33] Ørsted was not convinced he had obtained aluminium[34] and gave little importance to his discovery;[35] a different source suggests he was unable to continue his research for financial reasons.[8] As a result, and because he published his work in a Danish magazine unknown to the general European public, he is often not credited as the discoverer of the element;[34] some earlier sources went further and claimed Ørsted had not isolated aluminium.[36]
Berzelius attempted to isolate the metal in 1825 by carefully washing the potassium analog of the base salt in cryolite in a crucible. Prior to the experiment, he had correctly identified the formula of this salt as K3AlF6. He found no metal, but his experiment came very close to succeeding and was successfully reproduced many times later. Berzelius's mistake was in using an excess of potassium, which made the solution too alkaline; the alkaline solution dissolved all the newly formed aluminium.[37]
In 1827, German chemist Friedrich Wöhler repeated Ørsted's experiments but did not identify any aluminium. (Wöhler later wrote to Berzelius, "what Oersted assumed to be a lump of aluminium was certainly nothing but an aluminium-containing potassium".)[lower-alpha 6] He conducted a similar experiment, mixing anhydrous aluminium chloride with potassium, and produced a powder of aluminium.[32] He continued his research and in 1845 was able to produce small pieces of the metal and described some of its physical properties. Wöhler's description of the properties indicates that he had obtained impure aluminium.[39] Wöhler's—and other scientists'—failure to reproduce Ørsted's experiment only contributed to the non-recognition of Ørsted as the discoverer of aluminium, and Wöhler was credited as the discoverer following the success and descriptive details of his 1845 experiment.[40] The reason for the inconsistency between Ørsted's and Wöhler's experiments was only discovered in 1921 by Danish chemist Johan Fogh, who demonstrated that Ørsted's experiment was successful thanks to use of a large amount of excess aluminium chloride and an amalgam with low potassium content.[39]
Rare metal
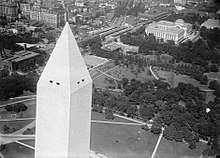
Since Wöhler's method could not yield large amounts of aluminium, the metal remained uncommon; its cost had exceeded that of gold before a new method was devised.[35]
French chemist Henri Étienne Sainte-Claire Deville announced an industrial method of aluminium production in 1854 at the Paris Academy of Sciences.[42] Aluminium chloride could be reduced by sodium, a metal more convenient and less expensive than potassium used by Wöhler.[43] Bars of aluminium were subsequently exhibited for the first time to the general public at the Exposition Universelle of 1855.[44] The metal was presented as "the silver from clay", and this name was soon widely used.[45] Napoleon III of France subsidized Deville's research, which cost about 20 times the annual income of an ordinary family.[46] Prior to the exposition, Napoleon is reputed to have held a banquet where the most honored guests were given aluminium utensils while others made do with gold.[35] From 1855 to 1859, the price of aluminium dropped by an order of magnitude, from US$500 to $40 per pound.[47] Even then, aluminium was still not of great purity and differed in properties by sample.[46]
In 1856, Deville and partners established the world's first industrial production of aluminium at a smelter in Rouen.[42] Deville's smelter moved in 1856–1857 to La Glacière, Nanterre, and finally to Salindres. The smelter was subsequently acquired by the French company Pechiney and the Compagnie d'Alais et de la Camargue, which later became world's largest in chemical production of aluminium. The factory's technology continued to improve, and the output of Salindres in 1872 exceeded that of Nanterre in 1857 by 900 times.[45] The factory in Salindres used bauxite as the primary aluminium ore;[48] some chemists, including Deville, sought to use cryolite, but with little success.[49] British engineer William Gerhard set up a plant with cryolite as the primary raw material in Battersea, London, in 1856, but technical and financial difficulties forced the closure of the plant in three years.[50]
Other chemists also sought to industrialize production of aluminium. British ironmaster Isaac Lowthian Bell produced aluminium from 1860 to 1874. During the opening of his factory, he waved to the crowd with a unique and costly aluminium top hat.[51] British engineer James Fern Webster launched the industrial production of aluminium by reduction with sodium in 1882; his aluminium was much purer than Deville's. Several other production sites were set up in the 1880s. All were made obsolete by electrolytic production.[52]
At the next fair in Paris in 1867, the visitors were presented with aluminium wire and foil; at the next fair in 1878, aluminium was considered the most important technological and scientific breakthrough.[53]
Electrolytic production
Aluminium was first synthesized electrolytically in 1854, independently by Deville and the German chemist Robert Wilhelm Bunsen. Their electrolysis methods did not become the basis for industrial production of aluminium because electrical supplies were inefficient at the time; this only changed with the invention of the dynamo by Belgian engineer Zénobe-Théophile Gramme in 1870 and the three-phase current by Russian engineer Mikhail Dolivo-Dobrovolsky in 1889.[54]
The first large-scale production method was independently developed by French engineer Paul Héroult and American engineer Charles Martin Hall in 1886; it is now known as the Hall–Héroult process. Electrolysis of pure alumina is impractical given its very high melting point; both Héroult and Hall realized its melting point could be significantly lowered by presence of molten cryolite. Héroult could not find enough interest in his invention as demand for aluminium was still small and the factory in Salindres did not wish to improve their process. In 1888, Héroult and his companions founded Aluminium Industrie Aktien Gesellschaft and started industrial production of aluminium bronze in Neuhausen am Rheinfall. This production was only active for a year, but during that time, Société électrométallurgique française was founded in Paris. The society purchased Héroult's patents and appointed him as the director of a smelter in Isère, which would produce aluminium bronze on a large scale at first and pure aluminium in a few months.[55][56]
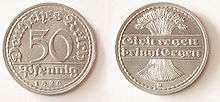
At the same time, Hall produced aluminium by the same process in his home at Oberlin,[57] and successfully tested it at the smelter in Lockport. He then sought to employ it for a large-scale production. For that, the smelter owners would have to radically change their production methods, which they were not willing to do because a mass production of aluminium would immediately drop the price of the metal. The president of the company considered purchasing Hall's patent to ensure that the competitors would not make use of the it. Hall founded the Pittsburgh Reduction Company in 1888 and initiated the mass production of aluminium. In the coming years, this technology was improved and new factories were constructed.[58]
The Hall–Héroult process converts alumina into the metal; Austrian chemist Carl Joseph Bayer discovered a way of purifying bauxite to yield alumina in 1889, now known as the Bayer process. Bayer sintered bauxite with alkali and leached it with water; after stirring the solution and introducing a seeding agent to it, he found a precipitate of pure aluminium hydroxide, which decomposed to alumina on heating. In a few years, he discovered that the aluminium contents of bauxite dissolved in the alkaline leftover from isolation of alumina solids; this was crucial for the industrial employment of this method.[59]
Modern production of the aluminium metal is based around the Bayer and Hall–Héroult processes. The Hall–Héroult process was further improved in 1920 by a team led by Swedish chemist Carl Wilhelm Söderberg. Previously, anode cells had been made from pre-baked coal blocks, which quickly corrupted and required replacement; the team introduced continuous electrodes made from a coke and tar paste in a reduction chamber. This greatly increased the world output of aluminium.[60]
Mass usage
Give me 30,000 tonnes of aluminium, and I will win the war.
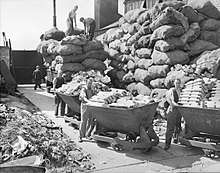
The prices for aluminium declined, and by the early 1890s, the metal had become widely used in jewelry, eyeglass frames, optical instruments, and many everyday items. Aluminium tableware began to be produced in the late 19th century and gradually supplanted copper and cast iron tableware in the first decades of the 20th century. Aluminium foil was popularized at that time. Aluminium is soft and light, but it was soon discovered that alloying it with other metals could increase its hardness while preserving low density. Aluminium alloys found many uses in the late 19th and early 20th centuries. For instance, aluminium bronze is applied to make flexible bands, sheets, and wire, and is widely employed in the shipbuilding and aviation industries.[63] Aviation used a new aluminium alloy, duralumin, invented in 1903.[64] Aluminium recycling started in the early 20th century and has been used extensively since[65] as aluminium is not impaired by recycling and thus can be recycled repeatedly.[66] At this point, only the metal that had not been used by end-consumers was recycled.[67] During World War I, major governments demanded large shipments of aluminium for light strong airframes. They often subsidized factories and the necessary electrical supply systems.[68][69] Overall production of aluminium peaked during the war: world production of aluminium in 1900 was 6,800 metric tons; in 1916, annual production exceeded 100,000 tons. The war created a greater demand for aluminium, which the growing primary production was unable to fully satisfy, and recycling grew intensely as well.[70] The peak in production was followed by a decline, then a swift growth.[67]
During the first half of the 20th century, the real price for aluminium continuously fell from $14,000 per metric ton in 1900 to $2,340 in 1948 (in 1998 United States dollars) with some exceptions such as the sharp price rise during World War I.[67] By the mid-20th century, aluminium had become a part of everyday lives, becoming an essential component of houseware.[71] Aluminium freight cars first appeared in 1931. Their lower mass allowed them to carry more cargo.[69] During the 1930s, aluminium emerged as a civil engineering material, being used in both basic construction and building interiors,[72] and advanced its use in military engineering for both airplanes and tank engines.[61]
Aluminium obtained from recycling was considered inferior to primary aluminium because of poorer chemistry control as well as poor removal of dross and slags. Recycling grew overall but largely depended on the output of primary production: for instance, as electric energy prices went down in the United States in the late 1930s, more primary aluminium could be produced in the energy-expensive Hall–Héroult process, rendering recycling less needed, and thus aluminium recycling rates went down.[70] By 1940, mass recycling of post-consumer aluminium had begun.[67]
During World War II, production peaked again, first exceeding 1,000,000 metric tons in 1941. The United Kingdom started an ambitious program of aluminium recycling; the Minister of Aircraft Production appealed to the public to donate any household aluminium for airplane building.[62] The Soviet Union received 328,000 metric tons of aluminium with the Lend-Lease policy;[73] this aluminium would be used in aircraft and tank engines.[74] Without these shipments, the output of the Soviet aircraft industry would have fallen by over a half.[75] Production fell after the war but then rose again.[67]
Exchange commodity
Earth's first artificial satellite, launched in 1957, consisted of two joined aluminium hemispheres, and almost all subsequent spacecraft have been made of aluminium.[60] The aluminium can was first manufactured in 1956 and employed as a container for drinks in 1958.[76] In the 1960s, aluminium was employed for production of wires and cables.[77] Since the 1970s, high-speed trains have commonly used aluminium for its lightness. For the same reason, the aluminium content of cars is growing.[69]
By 1955, the world market had been mostly divided by the Six Majors: Alcoa (successor of Hall's Pittsburgh Reduction Company), Alcan (originated as a part of that company), Reynolds, Kaiser, Pechiney (successor of Pechiney and the Compagnie d'Alais et de la Camargue that bought Deville's smelter), and Alusuisse (successor of Héroult's Aluminium Industrie Aktien Gesellschaft); their combined share of the market equaled 86%. From 1945, aluminium consumption grew by almost 10% each year for nearly three decades, gaining ground in building applications, electric cables, basic foils, and the aircraft industry. In the early 1970s, an additional boost came from the development of aluminium beverage cans.[78] The real price declined until the early 1970s because extraction and processing costs were lowered over technological progress and increased production of aluminium,[79] which first exceeded 10,000,000 metric tons in 1971.[67]
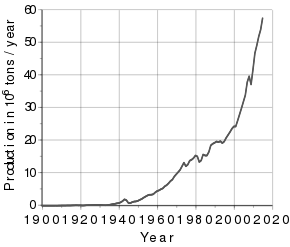
In the late 1960s, governments became aware of waste from the industrial production; they enforced a series of regulations favoring recycling and waste disposal. Söderberg anodes, more efficient but more harmful to the environment,[80] fell in disfavor, and production began to shift back to the pre-baked anodes.[81] The aluminium industry started to promote recycling of aluminium cans in an attempt to avoid restrictions on them.[70] This sparked recycling of aluminium previously used by end-consumers: for example, in the United States, levels of recycling of such aluminium increased 3.5 times from 1970 to 1980 and 7.5 times to 1990;[67] of all aluminium consumer products, cans remain the most important one.[82] Production costs for primary aluminium grew in the 1970s and 1980s, and this also contributed to the rise of aluminium recycling.[70]
In the 1970s, the increased demand for aluminium made it an exchange commodity; it entered the London Metal Exchange, world's oldest industrial metal exchange, in 1978.[60] Since then, aluminium has been traded for United States dollars and its price fluctuated along with the exchange rates of the currency.[83] The need to exploit lower-grade poorer quality deposits and the use of fast increasing input costs (above all, energy) increased the net cost of aluminium;[79] the real price began to grow in the 1970s.[84]
The increase of the real price and changes of tariffs and taxes started redistribution of the world producers' shares: the United States, the Soviet Union, and Japan accounted for nearly 60% of world's primary production in 1972 (and their combined share of consumption of primary aluminium was also close to 60%),[85] but their combined share only slightly exceeded 10% in 2012.[86] Production moved from the United States, Japan, and Western Europe to Australia, Canada, the Middle East, Russia, and China, where production was cheaper.[87] Production costs in the late 20th century changed because of advances in technology, lower energy prices, exchange rates of the United States dollar, and alumina prices.[88] The BRIC countries' combined share grew in the first decade of the 21st century from 32.6% to 56.5% in primary production and 21.4% to 47.8% in primary consumption.[89] China is accumulating an especially large share of world production, thanks to abundance of resources, cheap energy, and governmental stimuli;[90] it also increased its share of consumption from 2% in 1972 to 40% in 2010.[91] The only other country with a two-digit percentage was the United States with 11%; no other country exceeded 5%.[92] In the United States, Western Europe, and Japan, most aluminium was consumed in transportation, engineering, construction, and packaging.[92]
The world output continued to grow: in 2013, annual production of aluminium exceeded 50,000,000 metric tons. In 2015, it was record 57,500,000 tons.[67]
See also
Notes
- ↑ "One day a goldsmith in Rome was allowed to show the Emperor Tiberius a dinner plate of a new metal. The plate was very light, and almost as bright as silver. The goldsmith told the Emperor that he had made the metal from plain clay. He also assured the Emperor that only he, himself, and the Gods knew how to produce this metal from clay. The Emperor became very interested, and as a financial expert he was also a little concerned. The Emperor felt immediately, however, that all his treasures of gold and silver would decline in value if people started to produce this bright metal of clay. Therefore, instead of giving the goldsmith the regard expected, he ordered him to be beheaded."[5]
- ↑ Deville had established that heating a mixture of sodium chloride, clay, and charcoal yields numerous aluminium globules. This was published in the Proceedings of the Academy of Sciences but eventually forgotten.[6] French chemist André Duboin discovered that heating a mixture of borax, alumina, and smaller quantities of dichromate and silica in a crucible formed impure aluminium. Boric acid is abundant in Italy. This hints at the possibility that boric acid, potash, and clay under the reducing influence of coal may have produced aluminium in Rome.[6]
- ↑ Alumina was plentiful and could be reduced by coke in the presence of copper, giving aluminium–copper alloys. The Chinese did not have the technology to produce pure aluminium and the temperatures needed (around 2000 °C) were not achievable.[8] British sinologist and historian Joseph Needham did not suggest aluminium–copper alloys could have been produced that early but suggested it could have happened in medieval China.[9]
- ↑ "Today, I bring you the victory over the Turk. Every year they wring from the Christians more than three hundred thousand ducats for the alum with which we dye wool. For this is not found among the Latins except a very small quantity. [...] But I have found seven mountains so rich in this material that they could supply seven worlds. If you will give orders to engage workmen, build furnaces, and smelt the ore, you will provide all Europe with alum and the Turk will lose all his profits. Instead they will accrue to you..."[12]
- ↑ The terms "earth of alum" and "alumina" refer to the same substance. German-speaking authors used "earth of alum" (Alaun-Erde), while French authors used "alumina" (alumine).
- ↑ "Was Oersted für einen Aluminiumklumpen hielt, ist ganz gewiß nichts anderes gewesen als ein aluminiumhaltiges Kalium."[38]
References
- ↑ Greenwood, Norman N.; Earnshaw, Alan (1997). Chemistry of the Elements (2nd ed.). Butterworth-Heinemann. p. 217. ISBN 0-08-037941-9.
- ↑ Frank, W. B. (2009). "Aluminum". Ullmann's Encyclopedia of Industrial Chemistry. Wiley-VCH. doi:10.1002/14356007.a01_459.pub2. ISBN 978-3527306732.
- ↑ Drozdov 2007, p. 12.
- ↑ Drozdov 2007, pp. 12–14.
- ↑ Eskin, Dmitry (2008). Physical metallurgy of direct chill casting of aluminum alloys. CRC Press. p. 1. ISBN 978-1-4200-6282-3.
- 1 2 Duboin, A. (1902). "Les Romains ont-ils connu l'aluminium ?" [Did the Romans know about aluminum?]. La Revue scientifique (in French). 18 (24).
- ↑ Eggert, Gerhard (1995). "Ancient aluminum? Flexible glass? Looking for the real heart of a legend". Skeptical Inquirer. 19: 37–40. Retrieved 28 October 2017.
- 1 2 Butler, Anthony R.; Glidewell, Christopher; Pritchard, Sharee E. (1986). "Aluminium Objects from a Jin Dynasty Tomb – Can They Be Authentic?". Interdisciplinary Science Reviews. 11 (1): 88–94. doi:10.1179/isr.1986.11.1.88.
- ↑ Needham, Joseph (1974). Science and Civilisation in China. Volume 5: Chemistry and Chemical Technology, Part 2: Spagyrical Discovery and Invention: Magisteries of Gold and Immortality. Cambridge University Press. p. 193. ISBN 978-0-521-08571-7.
- ↑ Drozdov 2007, p. 16.
- ↑ Clapham, John Harold; Power, Eileen Edna (1941). The Cambridge Economic History of Europe: From the Decline of the Roman Empire. CUP Archive. p. 207. ISBN 978-0-521-08710-0.
- ↑ Setton, Kenneth Meyer (1976). The Papacy and the Levant, 1204-1571: The fifteenth century. American Philosophical Society. p. 239. ISBN 978-0-87169-127-9.
- 1 2 3 Drozdov 2007, p. 25.
- ↑ Weeks, Mary Elvira (1968). Discovery of the elements. 1 (7 ed.). Journal of chemical education. p. 187.
- 1 2 3 4 Richards 1896, p. 2.
- ↑ Drozdov 2007, p. 26.
- 1 2 Drozdov 2007, p. 27.
- ↑ Pott, Johann Heinrich (1746). Chymische Untersuchungen, welche fürnehmlich von der Lithogeognosia oder Erkäntniß und Bearbeitung der gemeinen einfacheren Steine und Erden ingleichen von Feuer und Licht handeln [Chemical investigations which primarily concern lithogeognosia or knowledge and processing of common simple rocks and earths [i.e., ores] as well as fire and light] (in German). 1. Voss, Christian Friedrich. p. 32.
- ↑ Chisholm, Hugh, ed. (1911). "Alum". Encyclopædia Britannica (11 ed.). Cambridge University Press.
- 1 2 Richards 1896, p. 3.
- ↑ Lennartson, Anders (2017). The Chemical Works of Carl Wilhelm Scheele. Springer International Publishing. p. 32. ISBN 978-3-319-58181-1.
- ↑ Wiegleb, Johann Christian (1790). Geschichte des wachsthums und der erfindungen in der chemie, in der neuern zeit. Nicolai, Christoph Friedrich. p. 357.
- ↑ Wurtz, Adolphe (1865). "An introduction to chemical philosophies, according to modern theories". Chemical News. Manufacturing chemists. 15: 99.
- 1 2 Drozdov 2007, p. 31.
- ↑ Richards 1896, pp. 3–4.
- 1 2 3 4 5 Richards 1896, p. 4.
- 1 2 Guyton, Louis-Bernard (1795). "Expériences comparatives sur les terres, pour déterminer leur fusibilité, leur manière de se comporter avec les flux salins ou vitreux, et l'action dissolvante qu'elles exercent réciproquement les unes sur les autres" [Comparative experiments on the earth, to determine their fusibility, their behavior with the saline or vitreous flows, and the dissolving action they exert on each other]. Journal de l'École polytechnique (in French). 3: 299. Retrieved 11 November 2017.
- 1 2 Davy, Humphry (1812). "Of metals; their primary compositions with other uncompounded bodies, and with each other". Elements of Chemical Philosophy: Part 1. 1. Bradford and Inskeep. p. 201.
- ↑ Davy, Humphry (1808). "Electro Chemical Researches, on the Decomposition of the Earths; with Observations on the Metals obtained from the alkaline Earths, and on the Amalgam procured from Ammonia". Philosophical Transactions of the Royal Society. Royal Society of London. 98: 353. doi:10.1098/rstl.1808.0023. Retrieved 10 December 2009.
- ↑ Quinion, Michael (2005). Port Out, Starboard Home: The Fascinating Stories We Tell About the words We Use. Penguin Books Limited. pp. 23–24. ISBN 978-0-14-190904-2.
- ↑ Royal Danish Academy of Sciences and Letters (1827). Det Kongelige Danske Videnskabernes Selskabs philosophiske og historiske afhandlinger [The philosophical and historical dissertations of the Royal Danish Science Society] (in Danish). Popp. pp. XXV–XXVI.
- 1 2 Wöhler, Friedrich (1827). "Ueber das Aluminium" [About the aluminium]. Annalen der Physik und Chemie. 2 (in German). 11: 146–161.
- ↑ Drozdov 2007, p. 36.
- 1 2 Fontani, Marco; Costa, Mariagrazia; Orna, Mary Virginia (2014). The Lost Elements: The Periodic Table's Shadow Side. Oxford University Press. p. 30. ISBN 978-0-19-938334-4.
- 1 2 3 Venetski, S. (1969). "'Silver' from clay". Metallurgist. 13 (7): 451–453. doi:10.1007/BF00741130.
- ↑ Larned, Josephus Nelson (1923). The New Larned History for Ready Reference, Reading and Research: The Actual Words of the World's Best Historians, Biographers and Specialists; a Complete System of History for All Uses, Extending to All Countries and Subjects and Representing the Better and Newer Literature of History. C.A. Nichols Publishing Company. p. 4472.
- ↑ Richards 1896, pp. 4–5.
- ↑ Bjerrum, Niels (1926). "Die Entdeckung des Aluminiums". Zeitschrift für Angewandte Chemie. 39 (9): 316–317. doi:10.1002/ange.19260390907. ISSN 0044-8249.
- 1 2 Drozdov 2007, p. 38.
- ↑ Light Metals: Aluminium, Magnesium and Titanium. 23. 1960. pp. 69–70.
- ↑ Binczewski, George J. (1995). "The Point of a Monument: A History of the Aluminum Cap of the Washington Monument". JOM. 47 (11): 20–25. doi:10.1007/bf03221302.
- 1 2 Drozdov 2007, p. 39.
- ↑ Sainte-Claire Deville, H. E. (1859). De l'aluminium, ses propriétés, sa fabrication [Aluminium, its properties, its manufacture] (in French). Paris: Mallet-Bachelier. Archived from the original on 30 April 2016.
- ↑ Karmarsch, C. (1864). "Fernerer Beitrag zur Geschichte des Aluminiums" [Further report on the history of aluminium]. Polytechnisches Journal (in German). 171 (1): 49.
- 1 2 Drozdov 2007, p. 41.
- 1 2 Drozdov 2007, p. 46.
- ↑ Polmear, Ian (2005). Light Alloys: From Traditional Alloys to Nanocrystals. Butterworth-Heinemann. p. 15. ISBN 978-0-08-049610-8.
- ↑ Drozdov 2007, p. 42.
- ↑ Drozdov 2007, pp. 43–45.
- ↑ McNeil 1990, p. 104.
- ↑ Howell, Georgina (2010). Gertrude Bell: Queen of the Desert, Shaper of Nations. Farrar, Straus and Giroux. pp. 4–5. ISBN 978-1-4299-3401-5.
- ↑ McNeil 1990, pp. 104–106.
- ↑ Drozdov 2007, p. 49.
- ↑ Drozdov 2007, pp. 52–53.
- ↑ Drozdov 2007, pp. 55–59.
- ↑ "Alusuisse" (in German). Historisches Lexikon der Schweiz. 2013. Retrieved 26 November 2017.
- ↑ Aluminum World. 1894. p. 66.
- ↑ Drozdov 2007, pp. 59–61.
- ↑ Drozdov 2007, p. 74.
- 1 2 3 "Aluminium history". All about aluminium. Retrieved 7 November 2017.
- 1 2 Drozdov 2007, p. 85.
- 1 2 Thorsheim, Peter (2015). Waste into Weapons. Cambridge University Press. pp. 66–69. ISBN 978-1-107-09935-7.
- ↑ Drozdov 2007, pp. 64–69.
- ↑ Drozdov 2007, pp. 66–69.
- ↑ Schlesinger, Mark E. (2013). Aluminum Recycling, Second Edition. CRC Press. pp. 2–6. ISBN 978-1-4665-7025-2.
- ↑ Lumley, Roger (2010). Fundamentals of Aluminium Metallurgy: Production, Processing and Applications. Elsevier Science. p. 70. ISBN 978-0-85709-025-6.
- 1 2 3 4 5 6 7 8 "Aluminum. Supply-Demand Statistics". Historical Statistics for Mineral Commodities in the United States (Report). United States Geological Survey. 2017. Retrieved 9 November 2017.
- ↑ Ingulstad, Mats (2012). "'We Want Aluminum, No Excuses': Business-Government Relations in the American Aluminum Industry, 1917–1957". In Ingulstad, Mats; Frøland, Hans Otto. From Warfare to Welfare: Business-Government Relations in the Aluminium Industry. Tapir Academic Press. pp. 33–68. ISBN 978-82-321-0049-1.
- 1 2 3 "Aluminium in transport". All about aluminium. Retrieved 26 November 2017.
- 1 2 3 4 Schlesinger, Mark E. (2013). Aluminum Recycling, Second Edition. CRC Press. pp. 2–6. ISBN 978-1-4665-7025-2.
- ↑ Drozdov 2007, pp. 69–70.
- ↑ Drozdov 2007, pp. 165–166.
- ↑ Tyson, Joseph Howard (2010). The Surreal Reich. iUniverse. p. 179. ISBN 978-1-4502-4019-2.
- ↑ Chandonnet, Fern (2007). Alaska at War, 1941-1945: The Forgotten War Remembered. University of Alaska Press. p. 338. ISBN 978-1-60223-135-1.
- ↑ Albert Loren Weeks (2004). Russia's Life-saver: Lend-lease Aid to the U.S.S.R. in World War II. Lexington Books. p. 135. ISBN 978-0-7391-0736-2.
- ↑ Drozdov 2007, p. 135.
- ↑ The Evolution of Aluminum Conductors Used for Building Wire and Cable (PDF) (Report). National Electrical Manufacturers Association. 2012. Retrieved 26 November 2017.
- ↑ Nappi 2013, pp. 5–6.
- 1 2 Nappi 2013, p. 9.
- ↑ Bockris, John (2013). Comprehensive Treatise of Electrochemistry: Electrochemical Processing. Springer Science & Business Media. ISBN 9781468437850.
- ↑ "The Søderberg cell technology Future challenges and possibilities". www.researchgate.net. Retrieved 2018-10-04.
- ↑ "Aluminum Scrap". www.themetalcasting.com. 2018. Retrieved 2018-09-20.
- ↑ Nappi 2013, pp. 12–13.
- ↑ Nappi 2013, pp. 9–10.
- ↑ Nappi 2013, p. 7.
- ↑ Nappi 2013, p. 3.
- ↑ Nappi 2013, p. 10.
- ↑ Nappi 2013, pp. 14–15.
- ↑ Nappi 2013, p. 17.
- ↑ Nappi 2013, p. 20.
- ↑ Nappi 2013, p. 22.
- 1 2 Nappi 2013, p. 23.
Bibliography
- Drozdov, Andrey (2007). Aluminium: The Thirteenth Element. RUSAL Library. ISBN 978-5-91523-002-5.
- McNeil, Ian, ed. (1990). An Encyclopaedia of the history of technology. Routledge. pp. 104–106. ISBN 978-0-415-01306-2.
- Nappi, Carmine (2013). The global aluminium industry 40 years from 1972 (PDF) (Report). International Aluminium Institute. Retrieved 10 November 2017.
- Richards, Joseph William (1896). Aluminium: Its history, occurrence, properties, metallurgy and applications, including its alloys (3 ed.). Henry Carey Baird & Co.