Digital polymerase chain reaction
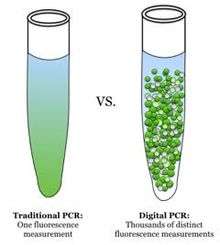
Digital polymerase chain reaction (digital PCR, DigitalPCR, dPCR, or dePCR) is a biotechnological refinement of conventional polymerase chain reaction methods that can be used to directly quantify and clonally amplify nucleic acids strands including DNA, cDNA or RNA. The key difference between dPCR and traditional PCR lies in the method of measuring nucleic acids amounts, with the former being a more precise method than PCR, though also more prone to error in the hands of inexperienced users.[1] A "digital" measurement quantitatively and discretely measures a certain variable, whereas an “analog” measurement extrapolates certain measurements based on measured patterns. PCR carries out one reaction per single sample. dPCR also carries out a single reaction within a sample, however the sample is separated into a large number of partitions and the reaction is carried out in each partition individually. This separation allows a more reliable collection and sensitive measurement of nucleic acid amounts. The method has been demonstrated as useful for studying variations in gene sequences — such as copy number variants and point mutations — and it is routinely used for clonal amplification of samples for next-generation sequencing.
Principles
The polymerase chain reaction method is used to quantify nucleic acids by amplifying a nucleic acid molecule with the enzyme DNA polymerase. Conventional PCR is based on the theory that amplification is exponential. Therefore, nucleic acids may be quantified by comparing the number of amplification cycles and amount of PCR end-product to those of a reference sample. However, many factors complicate this calculation, creating uncertainties and inaccuracies. These factors include the following: initial amplification cycles may not be exponential; PCR amplification eventually plateaus after an uncertain number of cycles; and low initial concentrations of target nucleic acid molecules may not amplify to detectable levels. However, the most significant limitation of PCR is that PCR amplification efficiency in a sample of interest may be different from that of reference samples. Since PCR is an exponential process, only twofold differences in amplification can be observed, greatly impacting the validity and precision of the results.
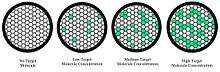
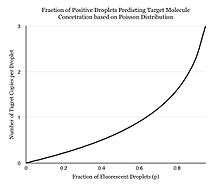
dPCR improves upon the current PCR practices by dividing up the reaction into multiple, smaller reactions. A sample is partitioned so that individual nucleic acid molecules within the sample are localized and concentrated within many separate regions. Micro well plates, capillaries, oil emulsion, and arrays of miniaturized chambers with nucleic acid binding surfaces can be used to partition the samples. A PCR solution is made similarly to a TaqMan assay, which consists of template DNA (or RNA), fluorescence-quencher probes, primers, and a PCR master mix, which contains DNA polymerase, dNTPs, MgCl2, and reaction buffers at optimal concentrations. The PCR solution is divided into smaller reactions and are then made to run PCR individually. After multiple PCR amplification cycles, the samples are checked for fluorescence with a binary readout of “0” or “1”. The fraction of fluorescing droplets is recorded. The partitioning of the sample allows one to estimate the number of different molecules by assuming that the molecule population follows the Poisson distribution, thus accounting for the possibility of multiple target molecules inhabiting a single droplet. Using Poisson's law of small numbers, the distribution of target molecule within the sample can be accurately approximated allowing for a quantification of the target strand in the PCR product.[2] Figure 2 shows the Poisson distribution of the copies of target molecule per droplet (CPD) based on the fraction of fluorescent droplets (p), represented by the function CPD=-ln(1-p).[3] This model simply predicts that as the number of samples containing at least one target molecule increases, the probability of the samples containing more than one target molecule increases. In conventional PCR, the number of PCR amplification cycles is proportional to the starting copy number. dPCR, however, is not dependent on the number of amplification cycles to determine the initial sample amount, eliminating the reliance on uncertain exponential data to quantify target nucleic acids and therefore provides absolute quantification.
The benefits of dPCR include increased precision through massive sample partitioning, which ensures reliable measurements in the desired DNA sequence due to reproducibility. With basic PCR, error rates are larger when detecting small fold-change differences, however, with dPCR the error rate decreases because smaller fold-change differences can be detected in DNA sequence. The technique itself reduces the use of a larger volume of reagent needed, which inevitably will lower experiment cost. Also, dPCR is highly quantitative as it does not rely on relative fluorescence of the solution to determine the amount of amplified target DNA.
Droplet Digital PCR
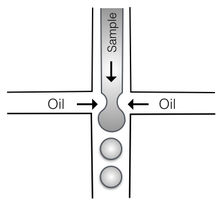
In Digital Droplet PCR (ddPCR) the PCR solution is divided into smaller reactions through a water oil emulsion technique, which are then made to run PCR individually. As shown in Figure 3, the PCR sample is partitioned into nanoliter-size samples and encapsulated into oil droplets.[4] The oil droplets are made using a droplet generator that applies a vacuum to each of the wells.[5] Approximately 20,000 oil droplets are made from each 20 μL sample.
Comparison between dPCR and Real-Time PCR (qPCR)
dPCR measures the actual number of molecules (target DNA) as each molecule is in one droplet, thus making it a discrete “digital” measurement. It provides absolute quantification because dPCR measures the positive fraction of samples, which is the number of droplets that are fluorescing due to proper amplification. This positive fraction accurately indicates the initial amount of template nucleic acid. Similarly, qPCR utilizes fluorescence; however, it measures the intensity of fluorescence at specific times (generally after every amplification cycle) to determine the relative amount of target molecule (DNA), but cannot specify the exact amount without constructing a standard curve using different amounts of a defined standard. It gives the threshold per cycle (CT) and the difference in CT is used to calculate the amount of initial nucleic acid. As such, qPCR is an analog measurement, which may not be as precise due to the extrapolation required to attain a measurement.[6] It should be noted that the previous reference was authored by employees of Bio-Rad, which provides commercial kits and reagents for dPCR, and therefore stands to profit from their promotion and sale.
dPCR measures the amount of DNA after amplification is complete and then determines the fraction of replicates. This is representative of an endpoint measurement as it requires the observation of the data after the experiment is completed. In contrast, qPCR records the relative fluorescence of the DNA at specific points during the amplification process, which requires stops in the experimental process. This “real-time” aspect of qPCR may theoretically affect results due to the stopping of the experiment. In practice, however, most qPCR thermal cyclers read each sample's fluorescence very quickly at the end of the annealing/extension step before proceeding to the next melting step, meaning this hypothetical concern is not actually relevant or applicable for the vast majority of researchers.
qPCR is unable to distinguish differences in gene expression or copy number variations that are smaller than twofold.[7] It is difficult to identify alleles with frequencies of less than 1% because highly abundant, common alleles would be matched with similar sequences. On the other hand, dPCR has been shown to detect a differences of less than 30% in gene expression, distinguish between copy number variations of that differ by only 1 copy, and identify alleles that occur at frequencies less than 0.1%.[8]
Applications
dPCR applications largely include, detection of mutant alleles; for example, dPCR can be used to quantitate mutant alleles in circulating tumor DNA. This technique can also be used for genetic prenatal diagnostics. In a research paper conducted by White et al., a dPCR assay was able to detect an L1 insertion event in as few as 0.01% to 0.1% of cells, which indicates the precision of this device.[9] dPCR has proved useful for quantitation of DNA methylation including the analysis of heterogeneous methylation.[10]
dPCR provides quantification of gene expression levels, especially with low-abundance miRNA due to the technique's sensitivity and precision. dPCR can be used for amplification of different types of RNA such as siRNA, mRNA, etc. Due to the high degree of cell-cell variation in gene expression, dPCR enable low copy number quantification in order to perform single cell analysis. Following variations among cells, dPCR enables an increased detection of differences in gene copy number in order to analyze complex behavioral traits, phenotypic variability, and diseases.[11]
Development & Commercialization of dPCR Methods
The first paper on dPCR was published by Dr Alec Morley and Pamela Sykes in 1992. The purpose was to quantify PCR targets in an attempt to track and measure the absolute lowest number of leukemic cells in a patient with leukemia. The purpose was to monitor residual disease in leukemia patients, and thereby treat the patients at the earliest possible moment of detection of disease recurrence. Further evolutions of the technology allowed for more widespread distribution of this technique, with small partitions created by emulsion droplets and/or microfluidics.[12]
In 1995, Brown at Cytonix and Silver National Institutes of Health coinvented single-step quantitization and sequencing methods employing nano-scale physical containment arrays (Brown, Silver),[13] and open chambers (Brown) using localized clonal colonies in 1D and 2D capillaries, macro volumes, gels, free chambers, and affinity surfaces/particles. resulting in a 1997 U. S. Patent,[14] and subsequent divisional and continuation patents. The concepts of electrowetting and digital microfluidics were further introduced (Brown) as one means of manipulating nano fluid volumes.
Digital PCR has been shown to be a possible surveillance tool for illnesses such as cancer.[15]
Vogelstein and Kinzler developed a technology called BEAMing based on digital PCR (Beads, Emulsion, Amplification, Magnetics) and quantified KRAS mutations in stool DNA from colorectal cancer patients.[16][17]
Dressman, et al., began using emulsion beads for digital PCR,.[18]
In 2006 Fluidigm introduced the first commercial system for digital PCR based on integrated fluidic circuits (chips) having integrated chambers and valves for partitioning samples.[19][20]
In 2008, Inostics started to provide BEAMing digital PCR services for the detection of mutations in plasma/serum and tissue.[21][22]
QuantaLife developed a different method of partitioning, called the Droplet Digital PCR (ddPCR) technology, which partitions a sample into approximately 20,000 droplets and digitally counts nucleic acid targets. In 2011, Bio-Rad Laboratories acquired Quantalife.[23]
In 2013, RainDance Technologies launched a digital PCR platform based on its picoliter-scale droplet technology, which generates up to 10 million picoliter-sized droplets per lane.[24][25] The technology was first demonstrated in a paper published in Lab on a Chip by scientists from Université de Strasbourg and Université Paris Descartes.[25][26] Later that year, RainDance Technologies announced a partnership with Integrated DNA Technologies to develop reagents for the digital PCR platform.[27] Bio-Rad Laboratories acquired RainDance Technologies in 2017 [28]
JnMedSys brought out their Clarity instrument in 2014 which partitioned samples into approximately 10,000 reactions for processing.[29]
Stilla Technologies commercially launched their Naica instrument in 2016 and was the first instrument to detect 3 colors per sample and partitioned their samples into approximately 30,000 droplets for processing.[30]
Digital PCR has many potential applications, including the detection and quantification of low-level pathogens, rare genetic sequences, copy number variations, and relative gene expression in single cells. This method provides the information with accuracy and precision.[31] Clonal amplification enabled by single-step digital PCR is a key factor in reducing the time and cost of many of the "next-generation sequencing" methods and hence enabling personal genomics.
MIQE
The "Minimum Information for Publication of Quantitative Digital PCR Experiments" or "digital MIQE" guidelines are a comprehensive set of best practices which aim to increase the validity and comparability of digital PCR experiments reported in published literature.[1] The guide was published in 2013 and followed publication in 2009 of "MIQE", a comparable guide for quantitative real-time PCR.[32][33] In the two years following publication of the "digital MIQE", less than 20% of published digital PCR papers have cited the guideline.[1]
References
- 1 2 3 Perkel J (May 2015). "Guiding our PCR experiments". BioTechniques. 58 (5): 217–21. doi:10.2144/000114283. PMID 25967899.
- ↑ Prediger E. "Digital PCR (dPCR)—What is it and why use it?". Integrated DNA Technologies.
- ↑ "Absolute Quantification of PCR Targets with the Droplet Digital™ PCR System". Bio-Rad. Retrieved 2018-05-26.
- ↑ "Droplet Digital™ PCR (ddPCR™) Technology". Bio-Rad. Retrieved 2018-05-26.
- ↑ Pinheiro LB, Coleman VA, Hindson CM, Herrmann J, Hindson BJ, Bhat S, Emslie KR (January 2012). "Evaluation of a droplet digital polymerase chain reaction format for DNA copy number quantification". Analytical Chemistry. 84 (2): 1003–11. doi:10.1021/ac202578x. PMC 3260738. PMID 22122760.
- ↑ Hindson BJ, Ness KD, Masquelier DA, Belgrader P, Heredia NJ, Makarewicz AJ, et al. (November 2011). "High-throughput droplet digital PCR system for absolute quantitation of DNA copy number". Analytical Chemistry. 83 (22): 8604–10. doi:10.1021/ac202028g. PMC 3216358. PMID 22035192.
- ↑ Ledger; et al. (2016). "Resolution of differences in gene expression using qPCR: a review of techniques". Journal of Biomolecular Technology. 42 (23): 230–245.
- ↑ Baker, Monya (2012-06-01). "Digital PCR hits its stride". Nature Methods. 9 (6): 541–544. doi:10.1038/nmeth.2027.
- ↑ White TB, McCoy AM, Streva VA, Fenrich J, Deininger PL (31 December 2014). "A droplet digital PCR detection method for rare L1 insertions in tumors". Mobile DNA. 5 (1): 30. doi:10.1186/s13100-014-0030-4. PMID 25598847.
- ↑ Mikeska T, Candiloro IL, Dobrovic A (August 2010). "The implications of heterogeneous DNA methylation for the accurate quantification of methylation". Epigenomics. 2 (4): 561–73. doi:10.2217/epi.10.32. PMID 22121974.
- ↑ "Droplet Digital PCR Applications Guide" (PDF). Bio-Rad. Retrieved 14 November 2016.
- ↑ Sykes PJ, Neoh SH, Brisco MJ, Hughes E, Condon J, Morley AA (September 1992). "Quantitation of targets for PCR by use of limiting dilution". BioTechniques. 13 (3): 444–9. PMID 1389177.
- ↑ Kalinina O, Lebedeva I, Brown J, Silver J (May 1997). "Nanoliter scale PCR with TaqMan detection". Nucleic Acids Research. 25 (10): 1999–2004. doi:10.1093/nar/25.10.1999. PMC 146692. PMID 9115368.
- ↑ US patent 6143496, "Method of sampling, amplifying and quantifying segment of nucleic acid, polymerase chain reaction assembly having nanoliter-sized sample chambers, and method of filling assembly", issued 2000-11-07, assigned to Cytonix and US Department of Health and Human Services
- ↑ Dong L, Meng Y, Sui Z, Wang J, Wu L, Fu B (August 2015). "Comparison of four digital PCR platforms for accurate quantification of DNA copy number of a certified plasmid DNA reference material". Scientific Reports. 5 (13174): 13174. doi:10.1038/srep13174. PMC 4548226. PMID 26302947.
- ↑ Vogelstein B, Kinzler KW (August 1999). "Digital PCR". Proceedings of the National Academy of Sciences of the United States of America. 96 (16): 9236–41. doi:10.1073/pnas.96.16.9236. PMC 17763. PMID 10430926.
- ↑ Pohl G, Shih I (January 2004). "Principle and applications of digital PCR". Expert Review of Molecular Diagnostics. 4 (1): 41–7. doi:10.1586/14737159.4.1.41. PMID 14711348.
- ↑ Dressman D, Yan H, Traverso G, Kinzler KW, Vogelstein B (July 2003). "Transforming single DNA molecules into fluorescent magnetic particles for detection and enumeration of genetic variations". Proceedings of the National Academy of Sciences of the United States of America. 100 (15): 8817–22. doi:10.1073/pnas.1133470100. PMC 166396. PMID 12857956.
- ↑ "digital PCR". digital-pcr.gene-quantification.info. Retrieved 2017-09-12.
- ↑ "Digital PCR Space Heating Up as Life Science Tool Vendors Begin Staking Claims". GenomeWeb. Retrieved 2017-09-12.
- ↑ SelectScience. "Inostics' BEAMing Digital PCR can Detect More Mutations Associated with Secondary Drug Resistance in GIST Patients than Testing Conventional Biopsies | SelectScience". www.selectscience.net. Retrieved 2017-09-12.
- ↑ "Bayer, Sysmex Inostics to Develop BEAMing CDx Tests for New Cancer Therapies". GenomeWeb. Retrieved 2017-09-12.
- ↑ "Bio-Rad Acquires QuantaLife and Digital PCR Technology" (Press release). Bio-Rad. 5 October 2011.
- ↑ "RainDance Launches Digital PCR Platform; Claims Sensitivity, Operating Cost Superiority". PCR Insider. Genomeweb. 29 March 2012.
- 1 2 Kubista, M. (1 May 2012). "Drivers and Hurdles for qPCR". Feature Article. Genetic Engineering & Biotechnology News. 32 (9).
- ↑ Pekin D, Skhiri Y, Baret JC, Le Corre D, Mazutis L, Salem CB, Millot F, El Harrak A, Hutchison JB, Larson JW, Link DR, Laurent-Puig P, Griffiths AD, Taly V (July 2011). "Quantitative and sensitive detection of rare mutations using droplet-based microfluidics". Lab on a Chip. 11 (13): 2156–66. doi:10.1039/c1lc20128j. PMID 21594292.
- ↑ "RainDance, IDT Partner on Consumables for Digital PCR Platform". GenomeWeb Daily News. Genomeweb. 15 October 2012.
- ↑ "Bio-Rad to Acquire RainDance Technologies". GenomeWeb. Retrieved 2018-07-05.
- ↑ "JN Medsys introduces Clarity™ Digital PCR system – JN Medsys". www.jnmedsys.com. Retrieved 2018-07-05.
- ↑ "Discover the Simplicity of Crystal Digital PCR with the Naica System". www.stillatechnologies.com. Retrieved 2018-07-05.
- ↑ Pinheiro LB, Coleman VA, Hindson CM, Herrmann J, Hindson BJ, Bhat S, Emslie KR (January 2012). "Evaluation of a droplet digital polymerase chain reaction format for DNA copy number quantification". Analytical Chemistry. 84 (2): 1003–11. doi:10.1021/ac202578x. PMC 3260738. PMID 22122760.
- ↑ Huggett JF, Foy CA, Benes V, Emslie K, Garson JA, Haynes R, Hellemans J, Kubista M, Mueller RD, Nolan T, Pfaffl MW, Shipley GL, Vandesompele J, Wittwer CT, Bustin SA (June 2013). "The digital MIQE guidelines: Minimum Information for Publication of Quantitative Digital PCR Experiments". Clinical Chemistry. 59 (6): 892–902. doi:10.1373/clinchem.2013.206375. PMID 23570709.
- ↑ Bustin SA, Benes V, Garson JA, Hellemans J, Huggett J, Kubista M, Mueller R, Nolan T, Pfaffl MW, Shipley GL, Vandesompele J, Wittwer CT (April 2009). "The MIQE guidelines: minimum information for publication of quantitative real-time PCR experiments". Clinical Chemistry. 55 (4): 611–22. doi:10.1373/clinchem.2008.112797. PMID 19246619.
External links
Reviews
- Brenan C, Morrison T (Autumn 2005). "High throughput, nanoliter quantitative PCR". Drug Discovery Today: Technologies. 2 (3): 247–53. doi:10.1016/j.ddtec.2005.08.017. PMID 24981943.
- Blow N (2007). "PCR's next frontier". Nature Methods. 4 (10): 869–875. doi:10.1038/nmeth1007-869.