Single-particle tracking
Single-particle tracking (SPT) is the observation of the motion of individual particles within a medium. The coordinates time series, which can be either in two dimensions (x, y) or in three dimensions (x, y, z), is referred to as a trajectory. The trajectory is typically analyzed using statistical methods to extract information about the underlying dynamics of the particle.[1][2][3] These dynamics can reveal information about the type of transport being observed (e.g., thermal or active), the medium where the particle is moving, and interactions with other particles. In the case of random motion, trajectory analysis can be used to measure the diffusion coefficient.
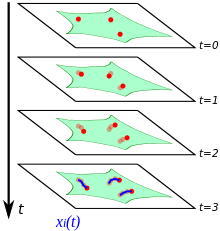
Applications
In life sciences, single-particle tracking is broadly used to quantify the dynamics of molecules/proteins in live cells (of bacteria, yeast, mammalian cells and live Drosophila embryos).[4][5][6][7] It has been extensively used to study the transcription factor dynamics in live cells.[8][9][10] Furthermore, exogenous particles are employed as probes to assess the mechanical properties of the medium, a technique known as passive microrheology.[11] This technique has been applied to investigate the motion of lipids and proteins within membranes,[12][13] molecules in the nucleus [14] and cytoplasm,[15] organelles and molecules therein,[16] lipid granules,[17][18][19] vesicles, and particles introduced in the cytoplasm or the nucleus. Additionally, single-particle tracking has been extensively used in the study of reconstituted lipid bilayers,[20] intermittent diffusion between 3D and either 2D (e.g., a membrane) [21] or 1D (e.g., a DNA polymer) phases, and synthetic entangled actin networks.[22][23]
Methods
The most common type of particles used in single particle tracking are based either on scatterers, such as polystyrene beads or gold nanoparticles that can be tracked using bright field illumination, or fluorescent particles. For fluorescent tags, there are many different options with their own advantages and disadvantages, including quantum dots, fluorescent proteins, organic fluorophores, and cyanine dyes.
On a fundamental level, once the images are obtained, single-particle tracking is a two step process. First the particles are detected and then the localized different particles are connected in order to obtain individual trajectories.
Besides performing particle tracking in 2D, there are several imaging modalities for 3D particle tracking, including multifocal plane microscopy,[24] double helix point spread function microscopy,[25] and introducing astigmatism via a cylindrical lens or adaptive optics.
Brownian diffusion
See also
References
- Metzler, Ralf; Jeon, Jae-Hyung; Cherstvy, Andrey G.; Barkai, Eli (2014). "Anomalous diffusion models and their properties: non-stationarity, non-ergodicity, and ageing at the centenary of single particle tracking". Phys. Chem. Chem. Phys. 16 (44): 24128–24164. Bibcode:2014PCCP...1624128M. doi:10.1039/c4cp03465a. ISSN 1463-9076. PMID 25297814.
- Manzo, Carlo; Garcia-Parajo, Maria F (2015-10-29). "A review of progress in single particle tracking: from methods to biophysical insights". Reports on Progress in Physics. 78 (12): 124601. Bibcode:2015RPPh...78l4601M. doi:10.1088/0034-4885/78/12/124601. ISSN 0034-4885. PMID 26511974.
- Anthony, Stephen; Zhang, Liangfang; Granick, Steve (2006). "Methods to Track Single-Molecule Trajectories". Langmuir. 22 (12): 5266–5272. doi:10.1021/la060244i. ISSN 0743-7463. PMID 16732651.
- Höfling, Felix; Franosch, Thomas (2013-03-12). "Anomalous transport in the crowded world of biological cells". Reports on Progress in Physics. 76 (4): 046602. arXiv:1301.6990. Bibcode:2013RPPh...76d6602H. doi:10.1088/0034-4885/76/4/046602. ISSN 0034-4885. PMID 23481518.
- Barkai, Eli; Garini, Yuval; Metzler, Ralf (2012). "Strange kinetics of single molecules in living cells". Physics Today. 65 (8): 29–35. Bibcode:2012PhT....65h..29B. doi:10.1063/pt.3.1677. ISSN 0031-9228.
- Mir, Mustafa; Reimer, Armando; Stadler, Michael; Tangara, Astou; Hansen, Anders S.; Hockemeyer, Dirk; Eisen, Michael B.; Garcia, Hernan; Darzacq, Xavier (2018), Lyubchenko, Yuri L. (ed.), "Single Molecule Imaging in Live Embryos Using Lattice Light-Sheet Microscopy", Nanoscale Imaging: Methods and Protocols, Methods in Molecular Biology, Springer New York, 1814, pp. 541–559, doi:10.1007/978-1-4939-8591-3_32, ISBN 978-1-4939-8591-3, PMC 6225527, PMID 29956254
- Ball, David A.; Mehta, Gunjan D.; Salomon-Kent, Ronit; Mazza, Davide; Morisaki, Tatsuya; Mueller, Florian; McNally, James G.; Karpova, Tatiana S. (2016-12-01). "Single molecule tracking of Ace1p in Saccharomyces cerevisiae defines a characteristic residence time for non-specific interactions of transcription factors with chromatin". Nucleic Acids Research. 44 (21): e160. doi:10.1093/nar/gkw744. ISSN 0305-1048. PMC 5137432. PMID 27566148.
- Mehta, Gunjan D.; Ball, David A.; Eriksson, Peter R.; Chereji, Razvan V.; Clark, David J.; McNally, James G.; Karpova, Tatiana S. (2018-12-06). "Single-Molecule Analysis Reveals Linked Cycles of RSC Chromatin Remodeling and Ace1p Transcription Factor Binding in Yeast". Molecular Cell. 72 (5): 875–887.e9. doi:10.1016/j.molcel.2018.09.009. ISSN 1097-2765. PMC 6289719. PMID 30318444.
- Morisaki, Tatsuya; Müller, Waltraud G.; Golob, Nicole; Mazza, Davide; McNally, James G. (2014-07-18). "Single-molecule analysis of transcription factor binding at transcription sites in live cells". Nature Communications. 5 (1): 4456. Bibcode:2014NatCo...5.4456M. doi:10.1038/ncomms5456. ISSN 2041-1723. PMC 4144071. PMID 25034201.
- Presman, Diego M.; Ball, David A.; Paakinaho, Ville; Grimm, Jonathan B.; Lavis, Luke D.; Karpova, Tatiana S.; Hager, Gordon L. (2017-07-01). "Quantifying transcription factor binding dynamics at the single-molecule level in live cells". Methods. The 4D Nucleome. 123: 76–88. doi:10.1016/j.ymeth.2017.03.014. hdl:11336/64420. ISSN 1046-2023. PMC 5522764. PMID 28315485.
- Wirtz, Denis (2009). "Particle-Tracking Microrheology of Living Cells: Principles and Applications". Annual Review of Biophysics. 38 (1): 301–326. CiteSeerX 10.1.1.295.9645. doi:10.1146/annurev.biophys.050708.133724. ISSN 1936-122X. PMID 19416071.
- Saxton, Michael J; Jacobson, Ken (1997). "Single-Particle Tracking: Applications to Membrane Dynamics". Annual Review of Biophysics and Biomolecular Structure. 26: 373–399. doi:10.1146/annurev.biophys.26.1.373. PMID 9241424.
- Krapf, Diego (2015), "Mechanisms Underlying Anomalous Diffusion in the Plasma Membrane", Lipid Domains, Current Topics in Membranes, 75, Elsevier, pp. 167–207, doi:10.1016/bs.ctm.2015.03.002, ISBN 9780128032954, PMID 26015283, retrieved 2018-08-20
- Ball, D. A; Mehta, G. D; Salomon-Kent, R; Mazza, D; Morisaki, T; Mueller, F; McNally, J. G; Karpova, T. S (2016). "Single molecule tracking of Ace1p in Saccharomyces cerevisiae defines a characteristic residence time for non-specific interactions of transcription factors with chromatin". Nucleic Acids Research. 44 (21): e160. doi:10.1093/nar/gkw744. PMC 5137432. PMID 27566148.
- Golding, Ido (2006). "Physical Nature of Bacterial Cytoplasm". Physical Review Letters. 96 (9): 098102. Bibcode:2006PhRvL..96i8102G. doi:10.1103/PhysRevLett.96.098102. PMID 16606319.
- Nixon-Abell, Jonathon; Obara, Christopher J.; Weigel, Aubrey V.; Li, Dong; Legant, Wesley R.; Xu, C. Shan; Pasolli, H. Amalia; Harvey, Kirsten; Hess, Harald F. (2016-10-28). "Increased spatiotemporal resolution reveals highly dynamic dense tubular matrices in the peripheral ER". Science. 354 (6311): aaf3928. doi:10.1126/science.aaf3928. ISSN 0036-8075. PMC 6528812. PMID 27789813.
- Tolić-Nørrelykke, Iva Marija (2004). "Anomalous Diffusion in Living Yeast Cells". Physical Review Letters. 93 (7): 078102. Bibcode:2004PhRvL..93g8102T. doi:10.1103/PhysRevLett.93.078102. PMID 15324280.
- Jeon, Jae-Hyung (2011). "In Vivo Anomalous Diffusion and Weak Ergodicity Breaking of Lipid Granules". Physical Review Letters. 106 (4): 048103. arXiv:1010.0347. Bibcode:2011PhRvL.106d8103J. doi:10.1103/PhysRevLett.106.048103. PMID 21405366.
- Chen, Yu; Rees, Thomas W; Ji, Liangnian; Chao, Hui (2018). "Mitochondrial dynamics tracking with iridium(III) complexes". Current Opinion in Chemical Biology. 43: 51–57. doi:10.1016/j.cbpa.2017.11.006. ISSN 1367-5931. PMID 29175532.
- Knight, Jefferson D.; Falke, Joseph J. (2009). "Single-Molecule Fluorescence Studies of a PH Domain: New Insights into the Membrane Docking Reaction". Biophysical Journal. 96 (2): 566–582. Bibcode:2009BpJ....96..566K. doi:10.1016/j.bpj.2008.10.020. ISSN 0006-3495. PMC 2716689. PMID 19167305.
- Campagnola, Grace; Nepal, Kanti; Schroder, Bryce W.; Peersen, Olve B.; Krapf, Diego (2015-12-07). "Superdiffusive motion of membrane-targeting C2 domains". Scientific Reports. 5 (1): 17721. arXiv:1506.03795. Bibcode:2015NatSR...517721C. doi:10.1038/srep17721. ISSN 2045-2322. PMC 4671060. PMID 26639944.
- Wong, I. Y. (2004). "Anomalous Diffusion Probes Microstructure Dynamics of Entangled F-Actin Networks". Physical Review Letters. 92 (17): 178101. Bibcode:2004PhRvL..92q8101W. doi:10.1103/PhysRevLett.92.178101. PMID 15169197.
- Wang, Bo; Anthony, Stephen M.; Bae, Sung Chul; Granick, Steve (2009-09-08). "Anomalous yet Brownian". Proceedings of the National Academy of Sciences. 106 (36): 15160–15164. Bibcode:2009PNAS..10615160W. doi:10.1073/pnas.0903554106. PMC 2776241. PMID 19666495.
- Ram, Sripad; Prabhat, Prashant; Chao, Jerry; Sally Ward, E.; Ober, Raimund J. (2008). "High accuracy 3D quantum dotifocal plane microscopy for the study of fast intracellular dynamics in live cells". Biophysical Journal. 95 (12): 6025–6043. Bibcode:2008BpJ....95.6025R. doi:10.1529/biophysj.108.140392. PMC 2599831. PMID 18835896.
- Badieirostami, M.; Lew, M. D.; Thompson, M. A.; Moerner, W. E. (2010). "Three-dimensional localization precision of the double-helix point spread function versus astigmatism and biplane". Applied Physics Letters. 97 (16): 161103. Bibcode:2010ApPhL..97p1103B. doi:10.1063/1.3499652. PMC 2980550. PMID 21079725.