Respiratory droplet
A respiratory droplet is a small aqueous droplet produced by exhalation, consisting of saliva or mucus and other matter derived from respiratory tract surfaces. Droplet sizes range from <5 µm to 1000 µm. Large droplets (larger than about 100 µm, but depending on conditions) fall to the ground or another surface before drying, but smaller ones fall slowly and dry so quickly that they usually become aerosolized particles. Respiratory droplets can be produced naturally as a result of breathing, talking, sneezing, coughing, or vomiting, or can be artificially generated through aerosol-generating medical procedures.[2] Since droplets can contain infectious bacterial cells or virus particles they are important factors in the transmission of respiratory diseases.
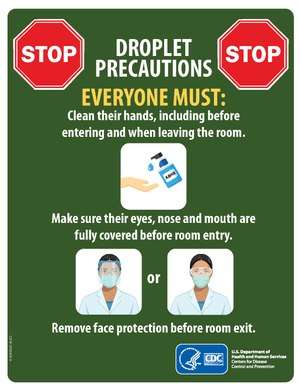
Description
Respiratory droplets from humans include various cells types (e.g. epithelial cells and cells of the immune system), physiological electrolytes contained in mucous and saliva (e.g. Na+, K+, Cl−), and, potentially, various pathogens.[2]
Droplets that dry in the air become droplet nuclei which float as aerosols and can remain suspended in air for considerable periods of time.[2]
Formation
Respiratory droplets can be produced in many ways. They can be produced naturally as a result of breathing, talking, sneezing, coughing, or singing. They can also be artificially generated in a healthcare setting through aerosol-generating procedures such as intubation, cardiopulmonary resuscitation (CPR), bronchoscopy, surgery, and autopsy.[2] Similar droplets may be formed through vomiting, flushing toilets, wet‐cleaning surfaces, showering or using tap water, or spraying graywater for agricultural purposes.[3]
Depending on the method of formation, respiratory droplets may also contain salts, cells, and virus particles.[2] In the case of naturally produced droplets, they can originate from different locations in the respiratory tract, which may affect their content.[3] There may also be differences between healthy and diseased individuals in their mucus content, quantity, and viscosity that affects droplet formation.[4]
Transport
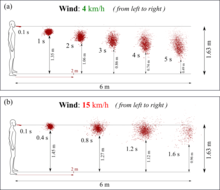
Different methods of formation create droplets of different size and initial speed, which affect their transport and fate in the air. As described by the Wells curve, the largest droplets fall sufficiently fast that they usually settle to the ground or another surface before drying out, and droplets smaller than 100 μm will rapidly dry out,before settling on a surface.[2][3] Once dry, they become solid droplet nuclei consisting of the non-volatile matter initially in the droplet. Respiratory droplets can also interact with other particles of non-biological origin in the air, which are more numerous than them.[3] When people are in close contact, liquid droplets produced by one person may be inhaled by another person; droplets larger than 10 μm tend to remain trapped in the nose and throat while smaller droplets will penetrate to the lower respiratory system.[4]
Advanced Computational Fluid Dynamics showed that at wind speeds varying from 4 to 15 km/h, respiratory droplets may travel up to 6 meters. [5][6]
Role in disease transmission
A common form of disease transmission is by way of respiratory droplets, generated by coughing, sneezing, or talking. Respiratory droplet transmission is the usual route for respiratory infections. Transmission can occur when respiratory droplets reach susceptible mucosal surfaces, such as in the eyes, nose or mouth. This can also happen indirectly via contact with contaminated surfaces when hands then touch the face. Respiratory droplets are large and cannot remain suspended in the air for long, and are usually dispersed over short distances.[7]
Viruses spread by droplet transmission include influenza virus, rhinovirus, respiratory syncytial virus, enterovirus, and norovirus;[8] measles morbillivirus;[9] and coronaviruses such as SARS coronavirus (SARS-CoV-1)[8][9] and SARS-CoV-2 that causes COVID-19.[10][11] Bacterial and fungal infection agents may also be transmitted by respiratory droplets.[2] By contrast, a limited number of diseases can be spread through airborne transmission after the respiratory droplet dries out.[9]
Ambient temperature and humidity affect the survivability of bioaerosols because as the droplet evaporates and becomes smaller, it provides less protection for the infectious agents it may contain. In general, viruses with a lipid envelope are more stable in dry air, while those without an envelope are more stable in moist air. Viruses are also generally more stable at low air temperatures.[3]
Hazard controls
In a healthcare setting, droplet precautions include housing a patient in an individual room, limiting their transport outside the room and using proper personal protective equipment.[1][12] Droplet precautions are one of three categories of transmission-based precautions that are used in addition to standard precautions based on the type of infection a patient has; the other two are contact precautions and airborne precautions.[1] However, aerosol-generating procedures may produce smaller droplets that travel farther, and so droplet precautions may be insufficient when such procedures are performed.[13]
In general, higher ventilation rates can be used as a hazard control to dilute and remove respiratory particles. However, if unfiltered or insufficiently filtered air is exhausted to another location, it can lead to spreading of an infection.[3]
Surgical masks can be used to prevent droplet transmission, both for infected patients[1][12] and healthcare personnel.[1][14] It has been noted that during the 2002–2004 SARS outbreak, use of surgical masks and N95 respirators tended to decrease infections of healthcare workers.[13] While surgical masks create a physical barrier between the mouth and nose of the wearer and potential contaminants such as splashes and respiratory droplets, they are not designed to filter or block very small particles such as those that transmit airborne diseases because of the loose fit between the face mask and the face.[15]
History
German bacteriologist Carl Flügge in 1899 was the first to show that microorganisms in droplets expelled from the respiratory tract are a means of disease transmission. In the early 20th century, the term Flügge droplet was sometimes used for particles that are large enough to not completely dry out, roughly those larger than 100 μm.[16]
Flügge's concept of droplets as primary source and vector for respiratory transmission of diseases prevailed into the 1930s until William F. Wells differentiated between large and small droplets.[6][17] He developed the Wells curve, which describes how the size of respiratory droplets influences their fate and thus their ability to transmit disease.[18]
See also
References
- "Transmission-Based Precautions". U.S. Centers for Disease Control and Prevention. 2016-01-07. Retrieved 2020-03-31.
- Atkinson, James; Chartier, Yves; Pessoa-Silva, Carmen Lúcia; Jensen, Paul; Li, Yuguo; Seto, Wing-Hong (2009). "Annex C: Respiratory droplets". Natural Ventilation for Infection Control in Health-Care Settings. World Health Organization. ISBN 978-92-4-154785-7.
- Morawska, L. (2006-10-01). "Droplet fate in indoor environments, or can we prevent the spread of infection?" (PDF). Indoor Air. 16 (5): 335–347. doi:10.1111/j.1600-0668.2006.00432.x. ISSN 0905-6947. PMID 16948710.
- Gralton, Jan; Tovey, Euan; McLaws, Mary-Louise; Rawlinson, William D. (2011-01-01). "The role of particle size in aerosolised pathogen transmission: A review". Journal of Infection. 62 (1): 1–13. doi:10.1016/j.jinf.2010.11.010. PMC 7112663. PMID 21094184.
- Talib Dbouk and Dimitris Drikakis: On Coughing and Airborne Transmission to Humans. Physics of Fluids, 32, 053310, 19 May 2020.
- Wells, W. F. (1934). "On air-borne infection: study II. Droplets and droplet nuclei". American Journal of Epidemiology. 20 (3): 611–618. doi:10.1093/oxfordjournals.aje.a118097.
- "Clinical Educators Guide for the prevention and control of infection in healthcare". Australian National Health and Medical Research Council. 2010. p. 3. Archived (PDF) from the original on 2015-04-05. Retrieved 2015-09-12.
- La Rosa, Giuseppina; Fratini, Marta; Della Libera, Simonetta; Iaconelli, Marcello; Muscillo, Michele (2013-06-01). "Viral infections acquired indoors through airborne, droplet or contact transmission". Annali dell'Istituto Superiore di Sanità. 49 (2): 124–132. doi:10.4415/ANN_13_02_03. ISSN 0021-2571. PMID 23771256.
- "FAQ: Methods of Disease Transmission". Mount Sinai Hospital (Toronto). Retrieved 2020-03-31.
- Neeltjevan Doremalen, Dylan H.Morris, Myndi G.Holbrook et al.: Aerosol and Surface Stability of SARS-CoV-2 as Compared with SARS-CoV-1 The New England Journal of Medicine, April 2020.
- "Pass the message: Five steps to kicking out coronavirus". World Health Organization. 2020-02-23. Retrieved 2020-03-24.
- "Prevention of hospital-acquired infections" (PDF). World Health Organization (WHO). p. 45. Archived (PDF) from the original on 26 March 2020.
- Gamage, B; Moore, D; Copes, R; Yassi, A; Bryce, E (2005-03-01). "Protecting health care workers from SARS and other respiratory pathogens: A review of the infection control literature". American Journal of Infection Control. 33 (2): 114–121. doi:10.1016/j.ajic.2004.12.002. PMC 7132691. PMID 15761412.
- "Clinical Educators Guide: Australian Guidelines for the Prevention and Control of Infection in Healthcare". Australian National Health and Medical Research Council. December 2019. p. 20. Retrieved 2020-03-30.
- "N95 Respirators and Surgical Masks (Face Masks)". U.S. Food and Drug Administration. 2020-03-11. Retrieved 2020-03-28.
- Hare, R. (1964-03-01). "The transmission of respiratory infections". Proceedings of the Royal Society of Medicine. 57 (3): 221–230. doi:10.1177/003591576405700329. ISSN 0035-9157. PMC 1897886. PMID 14130877.
- Bourouiba, Lydia (2020-03-26). "Turbulent Gas Clouds and Respiratory Pathogen Emissions: Potential Implications for Reducing Transmission of COVID-19". JAMA. doi:10.1001/jama.2020.4756. ISSN 0098-7484. PMID 32215590.
- World Health Organization; Y. Chartier; C. L Pessoa-Silva (2009). Natural Ventilation for Infection Control in Health-care Settings. World Health Organization. p. 79. ISBN 978-92-4-154785-7.