Dehalogenation
Dehalogenation is a chemical reaction that involves the cleavage of C-Halogen bond to form product. Dehalogenation can be divided into two subclasses: reductive dehalogenation and hydro dehalogenation.
.png)
History
Organic halides belong to a class of organic compounds that contain carbon-halogen bond. In 1832, scientist named Justus von Liebig synthesized the first organic halide (charcoal) via chlorination of ethanol. Since then, organohalides have gained a lot of attention.[1] Organohalides are commonly used as pesticides, biodegradables, soil fumigants, refrigerants, chemical reagents – solvents, and polymers.[2][3][4] It has been classified as pollutant despite of their wide use in various applications. Due to which, dehalogenation is a key reaction to convert toxic organohalides to less hazardous products.
Rate of dehalogenation
Among halogens, fluorine is the most electronegative atom and will have the highest tendency to make the strongest bond with carbon. The rate of dehalogenation depends on the bond strength between carbon and halogen atom. The bond dissociation energies of carbon-halogen bonds are described as: H3C-I (234 KJmol-1), H3C-Br (293 KJmol-1), H3C-Cl (351 KJmol-1), and H3C-F (452 KJmol-1). Thus, for the same structures the bond dissociation rate for dehalogenation will be: F<< Cl < Br < I.[4] Additionally, the rate of dehalogenation for alkyl halide also varies with steric environment and follows this trend: primary > secondary > tertiary halides.[4]
Dehalogenation using various catalysts
The rate of dehalogenation differs with the type of substrate, metal’s oxidation state, and reducing agents used during the reaction.
Dehalogenation using alkali and alkaline-earth metals
Alkali and alkaline earth metals such as lithium, sodium, potassium, magnesium, and calcium have proven to be great dehalogenation catalysts. During dehalogenation reaction, the metals act as a reducing agent to break down carbon-halogen bonds. Halogen can then leave as a leaving group. The generic way to synthesize alkanes using alkali and alkaline-earth metal is shown in scheme 2:

Yus and his co-workers synthesized various lithium naphthalenide compounds that acts as a catalyst for lithiation of different functionalized halogenated arenes. The Li-arene reacted with water or deuterium to produce the dehalogenated product.[5][6][7] The major drawback of using lithium naphthalene catalysts is that it is hard to separate from the reaction mixture because naphthalene adsorbs on surface of arene substrates. In polymer chemistry, sodium metal has been used for dehalogenation process.[8][9] Removal of halogen atom from arene-halides in the presence of Grignard agent and water for the formation of new compound is known as Grignard degradation. Dehalogenation using Grignard reagents is a two steps hydrodehalogenation process. The reaction begins with the formation of alkyl/arene-magnesium-halogen compound, followed by addition of proton source to form dehalogenated product. Egorov and his co-workers have reported dehalogenation of benzyl halides using atomic magnesium in 3P state at 600°C. Toluene and bi-benzyls were produced as the product of the reaction.[10] Morisson and his co-workers also reported dehalogenation of organic halides by flash vacuum pyrolysis using magnesium.[11]
Dehalogenation using 1st row transition metal complexes
Many groups have reported dehalogenation processes using different forms of homogeneous and heterogeneous transition metal complexes such as 0M, metal-ligand complexes, metal salts, and metal supported on different supports.[12] Vanadium compounds in its low oxidation state tends to perform dehalogenation reaction via one electron reduction. The efficiency of the one-electron transfer system depends on the redox potential of both the vanadium complexes and the radicophiles.[13] Lithium chromium(I) dihydride[14] has also been used as a versatile reducing agent to dehalogenate various alkyl or aryl halides as shown in scheme 3:
_dihydride.png)
Other than these metals, iron is the most studied metal for the dehalogenation reaction. Cahiez and his co-workers reported reduction of bromo-alkenes using manganese(II) halide in presence of Fe(acac)3.[15] Inspired by this work, Mohammed and coworkers also performed hydrobromination of 1,1,-dibromo-1-alkenes in presence of Grignard agents and transition metals such as Fe and Cobalt.[16] Macromolecules such as Hematin, cobalamin, vitamin B12, and coenzyme F430 are also used for dichlorination of polychlorinated ethylenes and benzenes. Charles and his co-workers reported that Vitamin B12 and coenzyme F430 were capable of sequentially dechlorinating tetrachloroethylene to ethylene, while hematin was demonstrated to dechlorinate tetrachloroethylene to vinyl chloride.[17]
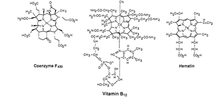
Javier and his-coworkers reported the synthesis of Iron (II) fluoride complexes. The complexes were then used as precursor and precatalysts for hydrodefluorination of fluorocarbons. They reported the first synthesis of a three-coordinate iron-fluoride complex.[18]
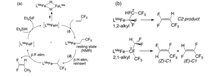
Jayant and his coworkers developed two phase systems for dehalogenation of trichloroethylenes. The kinetic model provides reaction process to take place in one phase while mass transfer between two phases.[19] There have been several reports on dehalogenation process performed using various other metals such as cobalt, nickel, palladium, silicon, and germanium.[20][2][21]
References
- Klein, U. Experiments, models, paper tools: Cultures of organic chemistry in the nineteenth century, Stanford university press: California, 2003, 191-193.
- Moon, J.; Lee, S. (2009). "Palladium catalyzed-dehalogenation of aryl chlorides and bromides using phosphite ligands". J. Organomet. Chem. 694 (3): 473–477. doi:10.1016/j.jorganchem.2008.10.052.
- Ware, G.; Gunther, F. Reviews of environmental contamination and toxicology, Springer-Verlag: New York, 1998, 155, 1-67.
- Trost, Barry M.; Fleming, Ian (1991). Comprehensive organic synthesis – selectivity, strategy and efficiency in modern organic chemistry. 1–9. Elsevier. pp. 793–809. ISBN 0080359299.
- Ramón, D.; Yus, M. Masked lithium bishomoenolates: Useful intermediates in organic synthesis, J. Org. Chem. 1991, 56, 3825-3831.
- Guijarro, A.; Ramón, D.; Yus, M. Naphthalene-catalysed lithiation of functionalized chloroarenes: regioselective preparation and reactivity of functionalized lithioarenes, Tetrahedron, 1993, 49, 469-482.
- Yus, M.; Ramón, D. Arene-catalysed lithiation reactions with lithium at low temperature, Chem. Comm. 1991, 398-400.
- Hawari, J. Regioselectivity of dechlorination: reductive dechlorination of polychlorobiphenyls by polymethylhydrosiloxane-alkali metal. J. Organomet. Chem. 1992, 437, 91-98.
- Mackenzie, K.; Kopinke, F.-D. Debromination of duroplastic flame-retarded polymers. Chemosphere, 1996, 33, 2423-2428.
- Tarakanova, A.; Anisimov, A.; Egorov, A. Low-temperature dehalogenation of benzyl halides with atomic magnesium in the 3P state. Russian Chemical Bulletin, 1999, 48, 147-151.
- Aitken, R.; Hodgson, P; Oyewale, A.’ Morrison, J. Dehalogenation of organic halides by flash vacuum pyrolysis over magnesium: a versatile synthetic method. Chem. Commun. 1997, 1163-1164.
- Grushin, V.; Alper, H. Activation of otherwise unreactive C-Cl bonds. Top. Organomet. Chem. 1999, 3, 193-226.
- Hirao, T.; Hirano, K.; Hasegawa, T.; Oshiro, Y.; Ikeda, I. A novel system consisting of low-valent vanadium and diethyl phosphonate or triethyl phosphite for the highly stereoselective reduction of gem-dibromocyclopropanes. J. Org. Chem. 1993, 58, 6529-6530.
- Eisch, J.; Alila, J. Lithium chromium(I) dihydride: a novel reagent for the versatile reductive metathesis, reductive cyclization, oligomerization, or polymerization of diverse organic substrates. Organometallics, 2000, 19, 1211-1213.
- Cahiez, G.; Benard, D.; Normant, J. Reduction des halogenures vinyliques et aryliques par les organomagnesiens en presence de manganese(II). J. organomet. Chem. 1976, 113, 107-113.
- Fakhfakh, M.; Franck, X.; Hocquemiller, R.’ Figadere, B. Iron catalyzed hydrodebromination of 2-aryl-1,1-dibromo-1-alkenes. J. Organomet. Chem. 2001, 624, 131-135.
- Gantzer, C.; Wackett, L. Reductive dichlorination catalyzed by bacterial transition-metal coenzymes. Environ. Sci. Technol. 1991, 25, 715-722.
- Vela, J.; Smith, J; Yu, Y.; Ketterer, N.; Flaschenriem, C.; Lachicotte, R.; Holland, P. Synthesis and reactivity of low-coordinate iron (II) fluoride complexes and their use in the catalytic hydrodefluorination of fluorocarbons. J. Am. Chem. Soc. 2005, 127, 7857-7870.
- Gotpagar, J.; Grulke, E.; Bhattacharyya, D.; Reductive dehalogenation of trichloroethylene: kinetic models and experimental verification. J. Hazardous mat. 1998, 62, 3, 243-264.
- Hetflejš, J.; Czakkoova, M.; Rericha, R.; Vcelak, J. Catalyzed dehalogenation of delor 103 by sodium hydridoaluminate. Chemosphere 2001, 44, 1521.
- Kagoshima, H.; Hashimoto, Y.; Oguro, D.; Kutsuna, T.; Saigo, K. Trophenylphosphine/germanium (IV) chloride combination: A new agent for the reduction of α-bromo carboxylic acid derivatives. Tetrahedron, 1998, 39, 1203-1206.