Ice algae

Ice algae are any of the various types of algal communities found in annual and multi-year sea or terrestrial ice. On sea ice in the polar oceans, ice algae communities play an important role in primary production[1]. The timing of blooms of the algae is especially important for supporting higher trophic levels at times of the year when light is low and ice cover still exists. Sea ice algal communities are mostly concentrated in the bottom layer of the ice, but can also occur in brine channels within the ice, in melt ponds, and on the surface.
Because terrestrial ice algae occur in freshwater systems, the species composition differs greatly from that of sea ice algae. These communities are significant in that they often change the color of glaciers and ice sheets, impacting the reflectivity of the ice itself.
Sea ice algae
Adapting to the sea ice environment
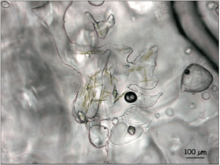
Microbial life in sea ice is extremely diverse[2][3][4], and includes abundant algae, bacteria and protozoa[5][6]. Algae in particular dominate the sympagic environment, with estimates of more than 1000 unicellular eukaryotes found to associate with sea ice in the Arctic[7][4][3][2]. Species composition and diversity vary based on location, ice type, and irradiance. In general, pennate diatoms such as Nitschia frigida (in the Arctic)[8] and Fragilariopsis cylindrus (in the Antarctic)[9] are abundant. Melosira arctica, which forms up to meter-long filaments attached to the bottom of the ice, are also widespread in the Arctic and are an important food source for marine species.[9]
While sea ice algae communities are found throughout the column of sea ice, abundance and community composition depends on the time of year[10]. There are many microhabitats available to algae on and within sea ice, and different algal groups have different preferences. For example, in late winter/early spring, motile diatoms like N. frigida have been found to dominate the uppermost layers of the ice, as far as briny channels reach, and their abundance is greater in multi-year ice (MYI) than in first year ice (FYI). Additionally, dinoflagellates have also been found to dominant in the early austral spring in Antarctic sea ice[5].
Sea ice algal communities can also thrive at the surface of the ice, in surface melt ponds, and in layers where rafting has occurred. In melt ponds, dominant algal types can vary with pond salinity, with higher concentrations of diatoms being found in melt ponds with higher salinity.[11] Because of their adaption to low light conditions, the presence of ice algae (in particular, vertical position in the ice pack) is primarily limited by nutrient availability. The highest concentrations are found at the base of the ice because the porosity of that ice enables nutrient infiltration from seawater.[12]
To survive in the harsh sea ice environment, organisms must be able to endure extreme variations in salinity, temperature, and solar radiation. Algae living in brine channels can secrete osmolytes, such as dimethylsulfoniopropionate (DMSP), which allows them to survive the high salinities in the channels after ice formation in the winter, as well as low salinities when the relatively fresh meltwater flushes the channels in the spring and summer.
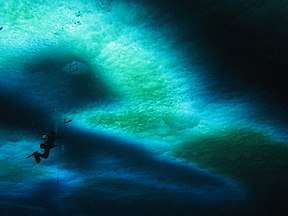
Some sea ice algae species secrete ice-binding proteins (IBP) as a gelatinous extracellular polymeric substance (EPS) to protect cell membranes from damage from ice crystal growth and freeze thaw cycles.[13] EPS alters the microstructure of the ice and creates further habitat for future blooms. Surface-dwelling algae produce special pigments to prevent damage from harsh ultraviolet radiation. Higher concentrations of xanthophyll pigments act as a sunscreen that protects ice algae from photodamage when they are exposed to damaging levels of ultraviolet radiation upon transition from ice to the water column during the spring.[3] Algae under thick ice have been reported to show some of the most extreme low light adaptations ever observed. Extreme efficiency in light utilization allows sea ice algae to build up biomass rapidly when light conditions improve at the onset of spring.[14]
Role in ecosystem
Ice algae play a critical role in primary production and serve as part of the base of the polar food web by converting carbon dioxide and inorganic nutrients to oxygen and organic matter through photosynthesis in the upper ocean of both the Arctic and Antarctic. Within the Arctic, estimates of the contribution of sea ice algae to total primary production ranges from 3-25%, up to 50-57% in high Arctic regions.[15][16] Sea ice algae accumulate biomass rapidly, often at the base of sea ice, and grow to form algal mats that are consumed by amphipods such as krill and copepods. Ultimately, these organisms are eaten by fish, whales, penguins, and dolphins.[14] When sea ice algal communities detach from the sea ice they are consumed by pelagic grazers, such as zooplankton, as they sink through the water column and by benthic invertebrates as they settle on the seafloor.[3] Sea ice algae as food are rich in polyunsaturated and other essential fatty acids, and are the exclusive producer of certain essential omega-3 fatty acids that are important for copepod egg production, egg hatching, and zooplankton growth and function.[3][17]
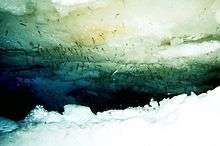
Temporal variation
The timing of sea ice algae blooms has a significant impact on the entire ecosystem. Initiation of the bloom is primarily controlled by the return of the sun in the spring (i.e. the solar angle). Because of this, ice algae blooms usually occurs before the blooms of pelagic phytoplankton, which require higher light levels and warmer water.[17] Early in the season, prior to the ice melt, sea ice algae constitute an important food source for higher trophic levels.[17] However, the total percentage that sea ice algae contribute to the primary production of a given ecosystem depends strongly on the extent of ice cover. The thickness of snow on the sea ice also affects the timing and size of the ice algae bloom by altering light transmission.[18] This sensitivity to ice and snow cover has the potential to cause a mismatch between predators and their food-source, sea ice algae, within the ecosystem. This so called match/mismatch has been applied to a variety of systems.[19] Examples have been seen in the relationship between zooplankton species, which rely on sea ice algae and phytoplankton for food, and juvenile walleye pollock in the Bering Sea.[20]
Bloom initialization
There are several ways in which sea ice algal blooms are thought to start their annual cycle, and hypotheses about these vary depending on water column depth, sea ice age, and taxonomic group. Where sea ice overlays deep ocean, it is proposed that cells trapped in multiyear ice brine pockets are reconnected to the water column below and quickly colonize nearby ice of all ages. This is known as the multiyear sea ice repository hypothesis[10]. This seeding source has been demonstrated in diatoms, which dominate sympagic blooms. Other groups, such as the dinoflagellates, which also bloom in the spring/summer, have been shown to maintain low cell numbers in the water column itself, and do not primarily overwinter within the ice[21]. Where sea ice covers ocean that is somewhat shallower, resuspension of cells from the sediment may occur[22].
Implications of climate change
Climate change and warming of Arctic and Antarctic regions have the potential to greatly alter ecosystem functioning. Decreasing ice cover in polar regions is expected to lessen the relative proportion of sea ice algae production to measures of annual primary production.[23][24] Thinning ice allows for greater production early in the season but early ice melting shortens the overall growing season of the sea ice algae. This melting also contributes to stratification of the water column that alters the availability of nutrients for algae growth by decreasing the depth of the surface mixed layer and inhibiting the upwelling of nutrients from deep waters. This is expected to cause an overall shift towards pelagic phytoplankton production.[24] Changes in multiyear ice volume[25] will also have an impact on ecosystem function in terms of bloom seeding source adjustment. Reduction in MYI, a temporal refugia for diatoms in particular, will likely alter sympagic community composition, resulting in bloom initialization that derives from species that overwinter in the water column or sediments instead[21].
Because sea ice algae are often the base of the food web, these alterations have implications for species of higher trophic levels.[15] The reproduction and migration cycles of many polar primary consumers are timed with the bloom of sea ice algae, meaning that a change in the timing or location of primary production could shift the distribution of prey populations necessary for significant keystone species. Production timing may also be altered by the melting through of surface melt ponds to the seawater below, which can alter sea ice algal habitat late in the growing season in such a way as to impact grazing communities as they approach winter[26].
The production of DMSP by sea ice algae also plays an important role in the carbon cycle. DMSP is oxidized by other plankton to dimethylsulfide (DMS), a compound which is linked to cloud formation. Because clouds impact precipitation and the amount of solar radiation reflected back to space (albedo), this process could create a positive feedback loop.[27] Cloud cover would increase the insolation reflected back to space by the atmosphere, potentially helping to cool the planet and support more polar habitats for sea ice algae. As of 1987, research has suggested that a doubling of cloud-condensation nuclei, of which DMS is one type, would be required to counteract warming due to increased atmospheric CO2 concentrations.[28]
Ice algae as a tracer for paleoclimate
Sea ice plays a major role in the global climate.[29] Satellite observations of sea ice extent date back only until the late 1970s, and longer term observational records are sporadic and of uncertain reliability.[30] While terrestrial ice paleoclimatology can be measured directly through ice cores, historical models of sea ice must rely on proxies.
Organisms dwelling on the sea ice eventually detach from the ice and fall through the water column, particularly when the sea ice melts. A portion of the material that reaches the seafloor is buried before it is consumed and is thus preserved in the sedimentary record.
There are a number of organisms whose value as proxies for the presence of sea ice has been investigated, including particular species of diatoms, dinoflagellate cysts, ostracods, and foraminifers. Variation in carbon and oxygen isotopes in a sediment core can also be used to make inferences about sea ice extent. Each proxy has advantages and disadvantages; for example, some diatom species that are unique to sea ice are very abundant in the sediment record, however, preservation efficiency can vary.[31]
Terrestrial ice algae
Algae also occur on terrestrial ice sheets and glaciers. The species found in these habitats are distinct from those associated with sea ice because the system is freshwater. Even within these habitats, there is a wide diversity of habitat types and algal assemblages. For example, cryosestic communities are specifically found on the surface of glaciers where the snow periodically melts during the day.[32] On enduring ice sheets and snow pack, terrestrial ice algae often color the ice due to accessory pigments, popularly known as "watermelon snow".
Implications for climate change
Recent research, known as the Black and Bloom project, has shown the impact of ice algae on the rate of melting of ice sheets. Because of the dark colors of the algae, sunlight absorption by the ice is enhanced, leading to an increase in the melting rate.[33] The goal of the Black and Bloom project is to determine how much the algae are contributing to the darkening of the ice sheets.
References
- ↑ Lee, Sang H.; Whitledge, Terry E.; Kang, Sung-Ho (December 2008). "Spring time production of bottom ice algae in the landfast sea ice zone at Barrow, Alaska". Journal of Experimental Marine Biology and Ecology. 367 (2): 204–212. doi:10.1016/j.jembe.2008.09.018. ISSN 0022-0981.
- 1 2 Rysgaard, S; Kühl, M; Glud, RN; Würgler Hansen, J (2001). "Biomass, production and horizontal patchiness of sea ice algae in a high-Arctic fjord (Young Sound, NE Greenland)". Marine Ecology Progress Series. 223: 15–26. doi:10.3354/meps223015.
- 1 2 3 4 5 Arrigo, Kevin R.; Brown, Zachary W.; Mills, Matthew M. (2014-07-15). "Sea ice algal biomass and physiology in the Amundsen Sea, Antarctica". Elem Sci Anth. 2: 000028. doi:10.12952/journal.elementa.000028. ISSN 2325-1026. Archived from the original on 2017-03-16.
- 1 2 Poulin, Michel; Daugbjerg, Niels; Gradinger, Rolf; Ilyash, Ludmila; Ratkova, Tatiana; Quillfeldt, Cecilie von (2011-03-01). "The pan-Arctic biodiversity of marine pelagic and sea-ice unicellular eukaryotes: a first-attempt assessment". Marine Biodiversity. 41 (1): 13–28. doi:10.1007/s12526-010-0058-8. ISSN 1867-1616.
- 1 2 Torstensson, Anders; Dinasquet, Julie; Chierici, Melissa; Fransson, Agneta; Riemann, Lasse; Wulff, Angela (2015-05-07). "Physicochemical control of bacterial and protist community composition and diversity in Antarctic sea ice". Environmental Microbiology. 17 (10): 3869–3881. doi:10.1111/1462-2920.12865. ISSN 1462-2912.
- ↑ Staley, James T.; Gosink, John J. (October 1999). "Poles Apart: Biodiversity and Biogeography of Sea Ice Bacteria". Annual Review of Microbiology. 53 (1): 189–215. doi:10.1146/annurev.micro.53.1.189. ISSN 0066-4227.
- ↑ Smoła, Z. T.; Kubiszyn, A. M.; Różańska, M.; Tatarek, A.; Wiktor, J. M. (2017-12-21), "Protists of Arctic Sea Ice", Interdisciplinary Approaches for Sustainable Development Goals, Springer International Publishing, pp. 133–146, ISBN 9783319717876, retrieved 2018-09-29
- ↑ Rozanska, M; Gosselin, M; Poulin, M; Wiktor, JM; Michel, C (2009-07-02). "Influence of environmental factors on the development of bottom ice protist communities during the winter–spring transition". Marine Ecology Progress Series. 386: 43–59. doi:10.3354/meps08092. ISSN 0171-8630. Archived from the original on 2017-03-17.
- 1 2 Vancoppenolle, Martin; Meiners, Klaus M.; Michel, Christine; Bopp, Laurent; Brabant, Frédéric; Carnat, Gauthier; Delille, Bruno; Lannuzel, Delphine; Madec, Gurvan (2013-11-01). "Role of sea ice in global biogeochemical cycles: emerging views and challenges". Quaternary Science Reviews. Sea Ice in the Paleoclimate System: the Challenge of Reconstructing Sea Ice from Proxies. 79: 207–230. doi:10.1016/j.quascirev.2013.04.011.
- 1 2 Olsen, Lasse M.; Laney, Samuel R.; Duarte, Pedro; Kauko, Hanna M.; Fernández-Méndez, Mar; Mundy, Christopher J.; Rösel, Anja; Meyer, Amelie; Itkin, Polona (July 2017). "The seeding of ice algal blooms in Arctic pack ice: The multiyear ice seed repository hypothesis". Journal of Geophysical Research: Biogeosciences. 122 (7): 1529–1548. doi:10.1002/2016jg003668. ISSN 2169-8953.
- ↑ Lee, Sang H.; Stockwell, Dean A.; Joo, Hyoung-Min; Son, Young Baek; Kang, Chang-Keun; Whitledge, Terry E. (2012-04-01). "Phytoplankton production from melting ponds on Arctic sea ice". Journal of Geophysical Research: Oceans. 117 (C4): C04030. doi:10.1029/2011JC007717. ISSN 2156-2202. Archived from the original on 2017-03-17.
- ↑ 1962-, Thomas, David N. (David Neville),. Sea ice. ISBN 9781118778388. OCLC 960106363.
- ↑ Krembs, Christopher; Eicken, Hajo; Deming, Jody W. (2011-03-01). "Exopolymer alteration of physical properties of sea ice and implications for ice habitability and biogeochemistry in a warmer Arctic". Proceedings of the National Academy of Sciences. 108 (9): 3653–3658. doi:10.1073/pnas.1100701108. ISSN 0027-8424. PMC 3048104. PMID 21368216. Archived from the original on 2015-12-28.
- 1 2 Mock, Thomas; Junge, Karen (2007-01-01). Seckbach, Dr Joseph, ed. Algae and Cyanobacteria in Extreme Environments. Cellular Origin, Life in Extreme Habitats and Astrobiology. Springer Netherlands. pp. 343–364. doi:10.1007/978-1-4020-6112-7_18. ISBN 9781402061110.
- 1 2 Kohlbach, Doreen; Graeve, Martin; A. Lange, Benjamin; David, Carmen; Peeken, Ilka; Flores, Hauke (2016-11-01). "The importance of ice algae-produced carbon in the central Arctic Ocean ecosystem: Food web relationships revealed by lipid and stable isotope analyses". Limnology and Oceanography. 61 (6): 2027–2044. doi:10.1002/lno.10351. ISSN 1939-5590. Archived from the original on 2017-03-15.
- ↑ Gosselin, Michel; Levasseur, Maurice; Wheeler, Patricia A.; Horner, Rita A.; Booth, Beatrice C. (1997). "New measurements of phytoplankton and ice algal production in the Arctic Ocean". Deep Sea Research Part II: Topical Studies in Oceanography. 44 (8): 1623–1644. doi:10.1016/s0967-0645(97)00054-4.
- 1 2 3 Leu, E.; Søreide, J. E.; Hessen, D. O.; Falk-Petersen, S.; Berge, J. (2011-07-01). "Consequences of changing sea-ice cover for primary and secondary producers in the European Arctic shelf seas: Timing, quantity, and quality". Progress in Oceanography. Arctic Marine Ecosystems in an Era of Rapid Climate Change. 90 (1–4): 18–32. doi:10.1016/j.pocean.2011.02.004.
- ↑ Mundy, C. J.; Barber, D. G.; Michel, C. (2005-12-01). "Variability of snow and ice thermal, physical and optical properties pertinent to sea ice algae biomass during spring". Journal of Marine Systems. 58 (3–4): 107–120. doi:10.1016/j.jmarsys.2005.07.003.
- ↑ Cushing, D (1990). "Plankton production and year-class strength in fish populations: An update of the match/mismatch hypothesis". Advances in Marine Biology. 26: 249–294.
- ↑ Siddon, Elizabeth Calvert; Kristiansen, Trond; Mueter, Franz J.; Holsman, Kirstin K.; Heintz, Ron A.; Farley, Edward V. (2013-12-31). "Spatial Match-Mismatch between Juvenile Fish and Prey Provides a Mechanism for Recruitment Variability across Contrasting Climate Conditions in the Eastern Bering Sea". PLOS One. 8 (12): e84526. doi:10.1371/journal.pone.0084526. ISSN 1932-6203. PMC 3877275. PMID 24391963. Archived from the original on 2017-03-15.
- 1 2 Kauko, Hanna M.; Olsen, Lasse M.; Duarte, Pedro; Peeken, Ilka; Granskog, Mats A.; Johnsen, Geir; Fernández-Méndez, Mar; Pavlov, Alexey K.; Mundy, Christopher J. (2018-06-06). "Algal Colonization of Young Arctic Sea Ice in Spring". Frontiers in Marine Science. 5. doi:10.3389/fmars.2018.00199. ISSN 2296-7745.
- ↑ Johnsen, Geir; Hegseth, ElseN�st (June 1991). "Photoadaptation of sea-ice microalgae in the Barents Sea". Polar Biology. 11 (3). doi:10.1007/bf00240206. ISSN 0722-4060. replacement character in
|first2=
at position 6 (help) - ↑ IPCC, 2007: Climate Change 2007: Synthesis Report. Contribution of Working Groups I, II and III to the Fourth Assessment Report of the Intergovernmental Panel on Climate Change [Core Writing Team, Pachauri, R.K and Reisinger, A. (eds.)]. IPCC, Geneva, Switzerland, 104 pp.
- 1 2 Lavoie, Diane; Denman, Kenneth L.; Macdonald, Robie W. (2010-04-01). "Effects of future climate change on primary productivity and export fluxes in the Beaufort Sea". Journal of Geophysical Research: Oceans. 115 (C4): C04018. doi:10.1029/2009JC005493. ISSN 2156-2202. Archived from the original on 2017-03-15.
- ↑ Nghiem, S. V.; Rigor, I. G.; Perovich, D. K.; Clemente-Colón, P.; Weatherly, J. W.; Neumann, G. (2007-10-04). "Rapid reduction of Arctic perennial sea ice". Geophysical Research Letters. 34 (19). doi:10.1029/2007gl031138. ISSN 0094-8276.
- ↑ Lee, Sang Heon; McRoy, C. Peter; Joo, Hyoung Min; Gradinger, Rolf; Cui, Huehua; Yun, Mi Sun; Chung, Kyung Ho; Kang, Sung-Ho; Kang, Chang-Keun (2011-09-01). "Holes in Progressively Thinning Arctic Sea Ice Lead to New Ice Algae Habitat". Oceanography. 24 (3): 302–308. doi:10.5670/oceanog.2011.81. ISSN 1042-8275.
- ↑ Sievert, Stefan; Kiene, Ronald; Schulz-Vogt, Heide (2007). "The Sulfur Cycle". Oceanography. 20 (2): 117–123. doi:10.5670/oceanog.2007.55.
- ↑ Charlson, Robert J.; Lovelock, James E.; Andreae, Meinrat O.; Warren, Stephen G. (1987-04-22). "Oceanic phytoplankton, atmospheric sulphur, cloud albedo and climate" (PDF). Nature. 326 (6114): 655–661. doi:10.1038/326655a0. ISSN 0028-0836.
- ↑ "All About Sea Ice | National Snow and Ice Data Center". nsidc.org. Archived from the original on 2017-03-20. Retrieved 2017-03-08.
- ↑ Halfar, Jochen; Adey, Walter H.; Kronz, Andreas; Hetzinger, Steffen; Edinger, Evan; Fitzhugh, William W. (2013-12-03). "Arctic sea-ice decline archived by multicentury annual-resolution record from crustose coralline algal proxy". Proceedings of the National Academy of Sciences. 110 (49): 19737–19741. doi:10.1073/pnas.1313775110. ISSN 0027-8424. PMC 3856805. PMID 24248344. Archived from the original on 2014-01-30.
- ↑ de Vernal, Anne; Gersonde, Rainer; Goosse, Hugues; Seidenkrantz, Marit-Solveig; Wolff, Eric W. (2013-11-01). "Sea ice in the paleoclimate system: the challenge of reconstructing sea ice from proxies – an introduction". Quaternary Science Reviews. Sea Ice in the Paleoclimate System: the Challenge of Reconstructing Sea Ice from Proxies. 79: 1–8. doi:10.1016/j.quascirev.2013.08.009.
- ↑ Komárek, Jiří; Nedbalová, Linda (2007-01-01). Seckbach, Dr Joseph, ed. Algae and Cyanobacteria in Extreme Environments. Cellular Origin, Life in Extreme Habitats and Astrobiology. Springer Netherlands. pp. 321–342. doi:10.1007/978-1-4020-6112-7_17. ISBN 9781402061110.
- ↑ Witze, Alexandra (2016-07-21). "Algae are melting away the Greenland ice sheet". Nature. 535 (7612): 336–336. doi:10.1038/nature.2016.20265. Archived from the original on 2017-03-15.
External links
- "Sea ice algae | ASU - Ask A Biologist". askabiologist.asu.edu. 2014-07-09. Retrieved 2017-03-15.
- "Algae". www.antarctica.gov.au. Retrieved 2017-03-15.
- "Snow algae". www.antarctica.gov.au. Retrieved 2017-03-15.
- "Ice algae: The engine of life in the central Arctic Ocean - AWI". www.awi.de. Retrieved 2017-03-15.
- "Sea Ice Algae is Staple of Arctic Food Chain". Live Science. Retrieved 2017-03-15.