Nucleic acid test
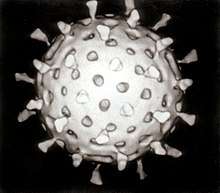
A nucleic acid test (NAT) or nucleic acid amplification test (NAAT) is a technique utilized to detect a particular nucleic acid, virus, or bacteria which acts as a pathogen in blood, tissue, urine, etc. The NAT system differs from other tests in that it detects genetic materials rather than antigens or antibodies. Detection of genetic materials allows an early diagnosis of a disease because the detection of antigens requires time for antigens to appear in the bloodstream.[1] Since the amount of a certain genetic material is usually very small, NAT includes an amplification step of the genetic material. There are several ways of amplification including polymerase chain reaction (PCR), strand displacement assay (SDA), or transcription mediated assay (TMA).[2] Virtually all nucleic acid amplification methods and detection technologies utilize the specificity of Watson-Crick base pairing; single-stranded probe or primer molecules capture DNA or RNA target molecules of complementary strands. Therefore, the design of probe strands is highly significant to raise the sensitivity and specificity of the detection. However, the mutants which forms the genetic basis for a variety of human diseases are usually slightly different from the normal nucleic acids. Often, they are only different in a single base, e.g., insertions, deletions, and single-nucleotide polymorphisms (SNPs). In this case, imperfect probe-target binding can easily occur which results in false-positive outcomes. Much research has been dedicated to achieving single-base specificity.
Breakthrough
In 2012, Yin’s research group has published a paper about optimizing the specificity of nucleic acid hybridization.[3] They introduced a ‘toehold exchange probe (PC)’ which consists of a pre-hybridized complement strand C and a protector strand P. Complement strand is longer than protector strand to have unbound tail in the end, a toehold. Complement is perfectly complementary with the target sequence. When the correct target(X) reacts with the toehold exchange probe(PC), P is released and hybridized product XC is formed. The standard free energy(∆) of the reaction is close to zero. On the other hand, if the toehold exchange probe(PC) reacts with spurious target(S), the reaction forwards, but the standard free energy increases to be less thermodynamically favorable. The standard free energy difference(∆∆) is significant enough to give obvious discrimination in yield. The discrimination factor Q is calculated as , the yield of correct target hybridization divided by the yield of spurious target hybridization. Through the experiments on different toehold exchange probes with 5 correct targets and 55 spurious targets with energetically representative single-base changes (replacements, deletions, and insertions), Yin’s group concluded that discrimination factors of these probes were between 3 and 100 + with the median 26. The probes function robustly from 10 °C to 37 °C, from 1 mM to 47 mM , and with nucleic acid concentrations from 1 nM to 5 M. They also figured out the toehold exchange probes work robustly even in RNA detection.
Further researches have been studied thereafter. In 2013, Seelig’s group published a paper about fluorescent molecular probes which also utilizes the toehold exchange reaction.[4] This enabled the optical detection of correct target and SNP target. They also succeeded in the detection of SNPs in E. coli-derived samples.
In 2015, David’s group achieved extremely high (1,000+) selectivity of single-nucleotide variants (SNVs) by introducing the system called ‘competitive compositions’.[5] In this system, they constructed a kinetic reaction model of the underlying hybridization processes to predict the optimal parameter values, which vary based on the sequences of SNV and wildtype (WT), on the design architecture of the probe and sink, and on the reagent concentrations and assay conditions. Their model succeeded in a median 890-fild selectivity for 44 cancer-related DNA SNVs, with a minimum of 200, which represents at least a 30-fold improvement over previous hybridization-based assays. In addition, they applied this technology to assay low VAF sequences from human genomic DNA following PCR, as well as directly to synthetic RNA sequences.
Based on the expertise, they developed a new PCR method called Blocker Displacement Amplification (BDA).[6] It is a temperature-robust PCR which selectively amplifies all sequence variants within a roughly 20 nt window by 1000-fold over wildtype sequences, allowing easy detection and quantitation of hundreds of potentials variants originally at ≤ 0.1% allele frequency. BDA achieve similar enrichment performance across anneal temperatures ranging from 56 °C to 64 °C. This temperature robustness facilitates multiplexed enrichment of many different variants across the genome, and furthermore enables the use of in- expensive and portable thermocycling instruments for rare DNA variant detection. BDA has been validated even on sample types including clinical cell-free DNA samples collected from the blood plasma of lung cancer patients.
Applications
References
- ↑ "What is the nucleic acid test (NAT)?". American Red Cross.
- ↑ Peter A. Leone, Joseph A. Duncan (2011). Tropical Infectious Diseases: Principles, Pathogens and Practice (Third Edition). Philadelphia: Elsevier. pp. 184–190.
- ↑ Peng Yin, David Zhang (2012). "Optimizing the specificity of nucleic acid hybridization". Nature Chemistry. 4: 208–214. doi:10.1038/NCHEM.1246. PMC 4238961.
- ↑ Georg Seelig, Sherry Chen (2013). "Conditionally fluorescent molecular probes for detecting single base changes in double-stranded DNA" (PDF). Nature Chemistry. 5: 782–789. doi:10.1038/NCHEM.1713. PMC 3844531.
- ↑ David Zhang, Juexiao Sherry Wang (2015). "Simulation-guided DNA probe design for consistently ultraspecific hybridization". Nature Chemistry. 7: 545–553. doi:10.1038/NCHEM.2266. PMC 4479422.
- ↑ David Zhang, Lucia R. Wu (2017). "Multiplexed enrichment of rare DNA variants via sequence-selective and temperature-robust amplification". Nature Biomedical Engineering. 1: 714–723. doi:10.1038/s41551-017-0126-5.
- ↑ Peter A. Leone, Joseph A. Duncan (2011). Tropical Infectious Diseases: Principles, Pathogens and Practice (Third Edition). Philadelphia: Elsevier. pp. 184–190.
- ↑ Molecular Medical Microbiology (Second Edition). Academic Press. 2015. pp. 1449–1469.
|first1=
missing|last1=
in Authors list (help) - ↑ Tuberculosis. Elsevier. 2009. pp. 738–745.
|first1=
missing|last1=
in Authors list (help) - ↑ Clinical Immunology (Fourth Edition). Elsevier. 2013. pp. 465–479.
|first1=
missing|last1=
in Authors list (help)